Research - (2022) Volume 12, Issue 11
Valorization of galacomannan of carob seeds (Ceratonia siliqua) by total heterogenous hydrolysis with protonated-kaolin and computer program simulation
S. Bouanani1,2* and A. Delimi2,3Abstract
This study aimed to valorize galactomannan (GM) isolated from Carob seeds (Ceratonia silica). Galactose and mannose were produced by the hydrolysis of GM with various amount of kaolin catalysts (0.005 to 0.05g) treated by tompans solutions (pH 4, 5.5, 7, and 9) and under different formique acid/THF reaction mixture molarity (1M, 2M, 8M, 14M and 22M). The different hydrolysis conditions were critical to affect the hydrolysis efficiency of galactomannan when compared to conventional hydrolysis in 2M TFA. The amounts of total reducing sugars(TRS) released was was determined using Somogyi-Nelson method and simulated by computer program. Actived kaolin (pH=4) effectively depolymerized galactomann with a maximum content of 36.075μg/ml total reducing sugar. However, the reaction mixture molarity 1M was more effective in galacomannan hydrolysis (34.75μg/mlTRS). Based on the fitting of the set of experimental data, we noticed that the model reproduced the evolution of the simple sugars content generated during the hydrolysis in most studied parameters.
Keywords
Galactomannan, Carob seeds, Total hydrolysis cinetic, Protonated-Kaolin, Computer simulation.
Introduction
The sugar industry has been interested for a long time in valorizing the products of the sugar beet industry. Various parietal polysaccharides have been the subject of valorization. Besides the sugar industry, new products are constantly appearing and underline the great richness of vegetable raw materials with this molecule. As an example, industrial preparation of carbohydrates from leguminous or cereal seeds (starch). The progress made in recent years in polysaccharide production technology has made it possible to develop products to meet the needs of consumers and industry. Successful utilization of biomass requires efficient conversion of the cellulosic portion to develop an integrated biorefinery to produce biofuel and chemicals economically. The utilization of lignocellulosic biomass depends on the level of success achieved in hydrolysis of the cellulose into its monomeric form, glucose (Ji et al., 2015; Kassaye et al., 2022). The polysaccharidic fraction of biomass must be effectively converted in order to construct an integrated biorefinery that can produce biofuel and chemicals at a reasonable cost. The degree of efficacy in hydrolyzing cellulose into its monomeric form, glucose, determines how effectively lignocellulosic biomass may be used.The hydrolysis of galacomannan carob seeds is carried out using a solid catalyst approach accompanied by a high glucose yield at moderate reaction conditions. Conventional chemical hydrolysis of polysaccharides requires a strong mineral acid at a high temperature with long contact times. It leads to a heterogeneous or homogeneous mixture of sugars, furan derivatives, and organic acids. It can lead to degradation and the formation of toxic compounds. Enzymatic hydrolysis has several disadvantages related to the high cost of enzymes, understanding of their mechanisms of action, limited polysaccharides conversion, and scalability challenges (Auxenfans et al., 2012). It is possible to modify the classical hydrolysis processes in order to make them cleaner and more efficient,safe and non-corrosive.For that, new strategies aim to avoid the use of solvents and strong mineral acids. Methodologies aimed at simplifying and making operations less polluting, as well as more resource- and energy-efficient, and thus the reactions on mineral solid supports (silicates, clays, and zeolites) (Kosinov et al., 2018; Onda et al., 2008, 2017). The use of solid acids keeps the advantages of using acid hydrolysis, but improves selectivity towards the glycosidic bonds at the expense of the dehydration of monosaccharides and can lead to higher sugar yield. Thus, solid acid catalysts represent an opportunity to develop more efficient and greener hydrolysis processes for soluble poly/oligosaccharides (Kassaye et al., 2016). The use of solid acids keeps the advantages of using acid hydrolysis, but improves selectivity towards the glycosidic bonds at the expense of the dehydration of monosaccharides and can lead to higher sugar yield. Thus, solid acid catalysts represent an opportunity to develop more efficient and greener hydrolysis processes for soluble poly/oligosaccharides (Vilcocq et al., 2014).As a result, attempts are underway to apply solid acid catalysts to obtain a total hydrolysis at temperatures less than 100°C and to convert lignocellulosic biomass to biochemicals compound. Clay minerals have been the subject of much research for several decades, in view of their varied industrial applications: ceramics, construction, and especially in the field of catalysis and ion exchange (Amacher et al., 1990; Dolcater et al., 1968).
Materials and Methods
Reagents and chemicals
All reagents were of analytical grade and used as purchased without further purification. 95% Tetrehydrofuran (THF) Panreac; 99% acide formique (Sigma); Trifluoroacetic acide (TFA), ethyl acetate, isopropanol, 72% sulfuric acid (H2SO4).
Materials
The kaolin was provided from Djebel Debagh Guelma. The feldspath collected from a quarry in the Edough massif (Wilaya de Annaba). The composition of the kaolin was carried out by X-rays diffraction (XRD) on a Siemens Instrument Difract AT V3.1. The X-ray source was monochromatic CuKα radiation, an accumulation time of 5 s/° with a resolution of 0.03°.Ceratonia siliqua pods were purchased from local markets in Annaba.The pods were separated, then the carob seeds were removed and were finely ground in a laboratory mill.The moisture content of the seeds was determined by drying the seeds up to a constant weight at 105°C (Nielsen, 2010). The Ash content was determined by dryin the seeds powder in oven for 5h at 600°C. The lipid fraction was extracted with n-hexane in a Soxhlet apparatus for 6h (ISO 659:2009). The monosacharide composition of GM was analysed by thin layer chtomatography (TLC).
Isolement and extraction of caroub seeds galactomannan
The polysaccharide extraction of carob seeds was performed with ethanol and distilled water as previously described (Bouzouita et al., 2007; Linden and Lorient, 1994).The extraction was performed without prior delipidation of the sample. The ground Ceratonia silca seeds were solubilized in distilled water under magnetic stirring for 24 hours. The supernatant was then treated with 96% ethanol or acetone after centrifugation. After filtration on Wattman filter paper, the precipitate was then dried in a desiccator or oven at 30°C.
Monosaccharide composition
0.1g of polysaccharides were introduced into a sealed tube, 1 ml of 72% H2SO4 was added. The reaction mixture was maintained for 20 min at room temperature and then 6 volumes of water were added. The hydrolysis reaction was continued in the oven at 100°C overnight. The solution was then cooled, neutralized with barium carbonate and filtered on glass fiber. The filtrate was deionized by passage on rhyolite (SiO2) (Al2O3) K2O or on celite (Labosi) (Adams, 1965). The monosccharides composition analysis was carried out by TLC in the solvent:ethyl acetate/isopropanol/water (65:23:12).then were revealed by aniline citrate. Potassium feldspath Al2O3 SiO2, K2O was used in place of celite after neutralization by BaCO3. This material has the same performance as celite.
Catalyst preparation
Kaolin was crushed and then sieved through a 60 mesh sieve and then through a textile material (valisier type). The retained fraction was used in our experiments. Kaolin (1g) were treated with buffer solutions (pH 4, 5.5, 7 and 9), dried in the oven at 50°C after one hour at room temperature. We increased the temperature by 10°C every 10 minutes until it reached 120°C. Finally, the catalyst was maintained for 16 hours at 120°C. Four kaolin catalysts were prepared KC4, KC5.5, KC7, KC9.
The effect of some parameters on the hydrolysis rate
Galactomannan 0.05 g were solubilized in 95% THF (Panreac) 5 ml. According to the experimental tests, the following reagents were added to the corresponding procedure:
A) Catalyst amount (g): kaolin catalyst (X g) was added and the reaction mixture was stirred with a rotary shaker for 4h at room temperature. The kaolin was remouved by filtration, the filtrate was analyzed by the Somogui-Nelson method (reducing extremity assay). Twenty experiments were conducted with a kaolin amounts ranging from 0.005g to 0.5g.
B) Catalyst treatment: Four catalysts were prepared by kaolin treatment with various buffer solutions (PH=4, 5.5, 7 and 9).
i. pH 4: 0.1M sodium acetate/0.1M formic acid tompan solution and a PH 4 formic acid solution;
ii. pH 5.5 and 7 prepared with sodium acetate/formic acid tompan solution;
iii. pH 9: Boric acid/Borax tompan solution.
C) Reaction mixture molarity: Formic acid solutions were prepared at different molarities (1M, 2M, 8M, 14M and 22M) in a 95% THF solution.
D) Hydrolysis temperature: The hydrolysis reaction was performed at different temperatures 30, 60, 100°C (without catalyst) then were compared with a classical hydrolysis (control) in 2M TFA at 100 °C.
Computer program was developed to simulate the galactomannan hydrolysis.
Catalytic reactions and kinetic analysis
Galactomann (GM) was subjected to totale hydrolysis by kaolin. In a preliminary study, hydrolysis of GM was performed in the absence of the catalyst at 100°C in 2M TFA/THF. In similar experimental work under identical conditions for the absence of a catalyst at various temperature (30°, 60 and 90°C). For a comparative study, the catalytic reactions were conducted in a in a round-bottom flask kept in an water bath under rotative stirring. The experimental process was as follows: 0.05 g of GM was dissolved in 5.0 mL 95% THF and then a catalytic amount of kaolin catalyst were added to the mixture. The reaction was continued for a desired time at a predetermined dissolution temperature. On the completion of the reaction time, the reaction mixture was quenched using an ice bath. Then, the catalyst and the hydrolysate were separated using vacuum filtration process. The hydrolysate was centrifuged at 10.000 rpm for 10 min and then stored in a refrigerator for further analysis.
Total hydrolysis by formic acid/trifluoroacetic acid (control)
The polysaccharide (0.05 g) were dissolved in 90% formic acid, the mixture was maintained 1 hour at 100°C. After evaporation of the acid, hydrolysis was continued in 2M trifluoroacetic acid (TFA) (Sigma) for 4 hours at 100°C. The acid was then removed by evaporation (Albersheim et al., 1967). TFA is a good choice for hydrolysis of neutral polysaccharides because it is volatile and can be removed by drying in a rotary evaporator or under a nitrogen flow. There are many studies on the hydrolysis of the different galactomannans with trifluoroacetic acid (TFA) under the following varying conditions: acid concentration (2-5 M), hydrolysis temperature (70-121°C), and hydrolysis time (1-8 h) (Liu et al., 2020).
Total hydrolysis with the kaolin catalyst
Experimental series were performed in order to study the effect of certain parameters on the hydrolysis rate of O-glycosidic bonds. Each series includes twenty (20) experiments with predetermined amounts of kaolin. GM (0.05 g) were solubilized, in tetrahydrofurane (5 ml), kaolin (previously treated or not) was added. The mixture was stirred by rotary stirring in a water bath for 4 hours at room temperature, in the presence or absence of formic acid. At the end of the reaction the catalyst was removed by filtration.
Hydrolysis product analysis
The hydrolysate samples were analyzed using arsenic-molybdic reagent in UV-Vis spectroscopy for total reducing sugar (TRS) yield with Somogyi-Nelson method at 700 nm. Based on the reducing properties of glucose in alkaline and hot media, the oxidizing reagent is a cuproalkaline reagent, made weakly alkaline by a mixture of carbonate and sodium hydrogen carbonate. When glucose is oxidized, copper oxide is formed, which was determined by colorimetry, by reducing an arsenic-molybdic reagent, with the formation of an intense blue complex, stable and soluble. A Calibration Range was prepared ranging from 0 to 80 g/ml (0, 10, 20, 30, and 40 μg/ml). The samples were heated in a boiling water bath for 20 minutes, then cooled in cold water bath for 5 minutes without stirring. Each tube received 2 ml of arsenomolybdic reagent, which was mixed by inverting and filled with 10 ml of cold boiled distilled water. The reading is done as soon as all outgassing has stopped against the blank, at 700 nm (Audigié et al., 1978). The resulting solution was cooled using an ice bath, and the analysis was carried out by measuring the absorbance of the sample (2 ml) using UV-Vis spectroscopy at 700 nm wavelength. The total reducing sugar Reactions yield was determined from the calibration curve formed using four-point concentration of standard glucose solutions.
Kinetic modeling of GM depolymerisation (Computer program with Matlab software)
The neuronal regression method (non linear regression between input and output) was applied for an estimation of the hydrolysis rate depending on some parameter.
R1=covariance function of Y (output): DOS, D4, D5, D7, D9 et DOM1, DOM2, DOM8, DOM14 DOM22.
R2=covariance function of U (input): PS=amount of catalyst
DOM was the absorbances measured for the different molarity.
DOS was the absorbances measured for every pH.
The identification of the program implies modeling the non linear system's behavior with the appropriate mathematical model; this model, which is frequently a simplification of the relationships between the system's various variables, must nevertheless continue to be representative of the studied system's behavior. Once the model equations were established, the objective was to determine the different parameters of the kinetic equations. This was made by the regression of the experimental data by Matlab software. In our case, the system studied was the polysaccharide hydrolysis by different catalyst amount with variation of the following parameters: pH tompan of the catalyst treatment solution and reaction mixture molarity. Our data are the measured absorbances for each parameter used to calculate reducing sugars. So we have one input (U) which is the concentration of the catalyst, and several outputs (Y) which were DOS, D4, D5, D7, D9, DOM, DOM2, DOM8, DOM14, and DOM22. In each example, the simulated results were shown as a continuous simulation line for each parameter under consideration, while the experimental data were shown as symbols.
Results and Discussion
Carob seed’s composition
Due to its positive effects on health and functional qualities, carob seed is becoming an increasingly popular ingredient in functional foods (Fidan et al., 2020). This study's objective was to assess the carob seed's composition of Carob seed had a moisture content (9.85 ± 0.07%), Ash content (1.9 ± 0.01%) and the lipid fraction (5.31 ± 0.02%) (Table 1), with mannose and galactose predominating among the monosaccharides (Fig. 1).
Sample | Moisture% (w/w) | Ash% (w/w) | Lipid% (w/w) |
---|---|---|---|
Carob seed | 9.85 ± 0.07 | 1.9 ± 0.01 | 5.31 ± 0.02 |
Table 1. Carob seed’s composition.
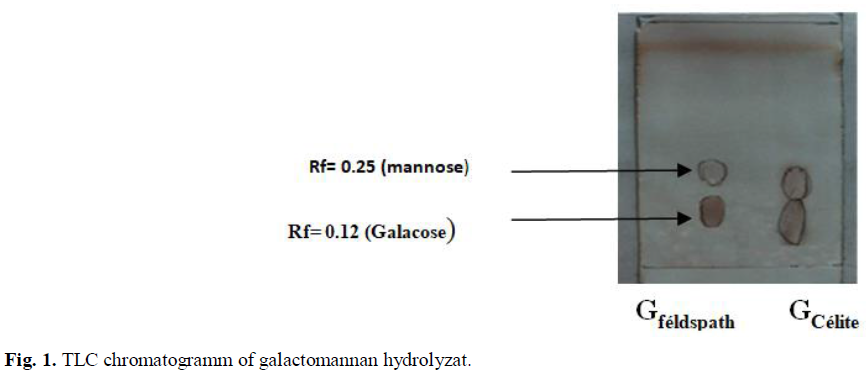
Fig 1: TLC chromatogramm of galactomannan hydrolyzat.
Dakia et al., (2007) reported that the composition of the carob seed includes approximatively 0.4% sucrose, 0.1% D-glucose, fructose, 0.1% starch, and a total phenols content of 0.661 mg/g. In the endosperm, the main polysaccharide is a galactomannan. The ratio mannose/galactose was 3.5 and 4 for isolated polysaccharide from carob seeds after purification with acetone (Fidan et al., 2020; Gillet, 2008). The M/G ratio of a galactomannan can vary within the carob galactomannans. It depends on the climatic conditions, the soil composition, the variety, the age of the tree and the growing conditions (Bouzouita et al., 2007; Dakia, 2009). The potassium feldspath Al2O3, SiO2, K2O was a material which presents the same performance as the celite commercialized by the company LABOSI. It needs to undergo preliminary work, such as an examination of the chemical elements present and the level of oxidation of specific elements, before it can be used commercially as a deionizer. The TLC chromatograms showed results of the galactomanan hydrolysates deionization with fedspath and celite (Fig. 1). Hydrolyzats treated with felspath showed a better separation of the two monosaccharides by Thin layer chromaography than those treated with celite.
Galactomannan is a plysaccahride which is soluble in water and. Most legume-seed galactomannans consist of linear chains of (1→4)-linked β-D-mannopyranosyl residues, to which are attached varying proportions of (1→6)-linked α-D-galactopyranosyl groups as single-unit side-chains (Dea et al., 1986). Afer totale hydrolysis of the galactmannan extrated, the TLC chromatogram showed two spot corresponded to galactose and mannaose with Rf of 0.12 and 0.25 respectively. Purified galactomanan represents between 98-99,1% of Galacose and mannose (Lopes da Silva and Gonçalves, 1990; McCleary et al., 1985).
Catalyst characterisation (XRD)
Mineral composition of kaolin was analysed with X ray diffraction (XRD) methods. The results were collected in Table 2.
SiO2 | Fe2O3 | Al2O3 | CaCO3 | MgO | PAF |
---|---|---|---|---|---|
45.10% | 0.55% | 36.66% | 1.35% | 0.05% | 15.24% |
Si/Al=1.23 |
Table 2. Chemical composition of the kaolin from Djebel Debegh–Guelma by XRD.
The effect of temperature on the yield of polysaccharide hydrolysis
Fig. 2 and Fig. 3 showed the effect of temperature on the yield of polysaccharide hydrolysis, which was significantly affected by reaction temperature and Kaolin treatment. The results of the hydrolysis reaction at various temperatures showed that 90°C produced the highest yield of TRS. The TRS yield showed an increase as the temperature was raised from 30°C to 90°C. The kaolin catalyst treated by tampon solution at pH 4 showed the most TRS content released (Fig. 2). However, the yield showed a decreasing trend as molarity of reactional mixture increased due to the decomposition of TRS (Fig. 3).
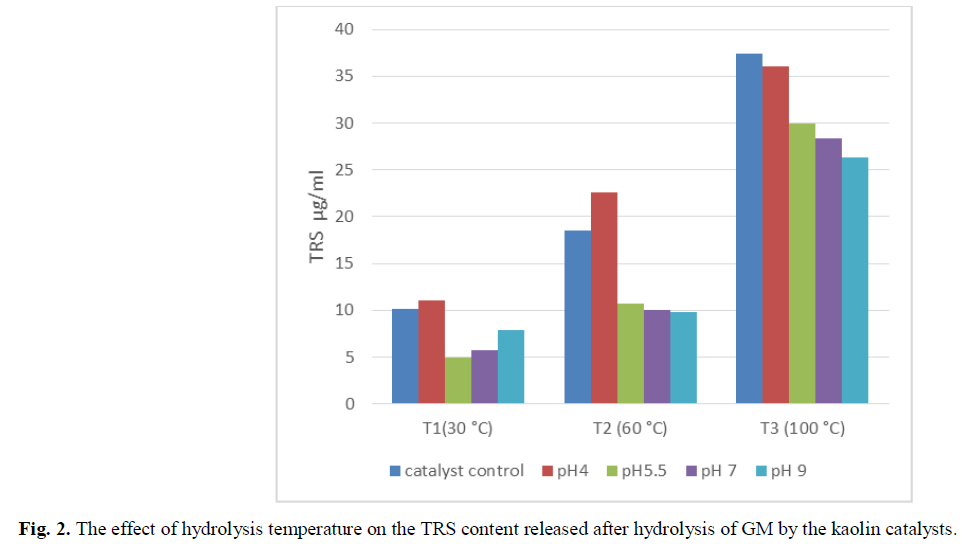
Fig 2: The effect of hydrolysis temperature on the TRS content released after hydrolysis of GM by the kaolin catalysts.
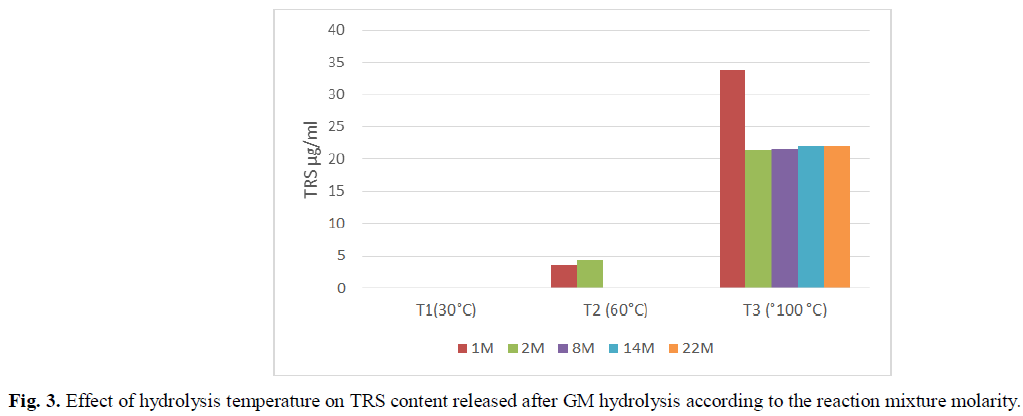
Fig 3: Effect of hydrolysis temperature on TRS content released after GM hydrolysis according to the reaction mixture molarity.
These findings agree with those found by Kusema et al., (2012), who reported that the hydrolysis rate was exclusively dependent on the pH of the solution but independent of the concentration and type of acid (Kusema et al., 2012). In comparison to homogeneous catalysts, heterogeneous acid catalysts are more effective for practical applications in large-scale production because they are easily recovered and have high production sustainability.
The mechanism of MG hydrolysis with the solid catalyst is like the hydrolysis of polysaccharide(celluloses) in the presence of homogeneous acidic catalysts such as sulfuric acids. The glycosidic bonds in the galactomannan are broken by Bronsted acid sites similar to H+ ions of acidic solutions to release the glucose monomers throug binding with the glycosidic oxygen atom, leading to hydrolysis of GM to simple sugars. H+ cations generated in situ from the Bronsted acid sites of catalyst are the key active species for the effective hydrolysis of GM by H+ catalyst (Kassaye et al., 2022).
Optimization of hydrolysis condition by programm computer simulation
Several studies have reported polysaccharide hydrolysis by heterogeneous catalysts (Huang and Fu, 2013; Onda et al., 2017; Sakamoto et al., 2020; Vilcocq et al., 2014; Zhang et al., 2013). Catalytic results showed that the kaolin catalysts KC4 was more effective than common acid catalysts (native kaolin) on GM hydrolysis. The following figures (Fig. 4, Fig. 5 and Fig. 6) showed the results of the optimization for the different experiments.
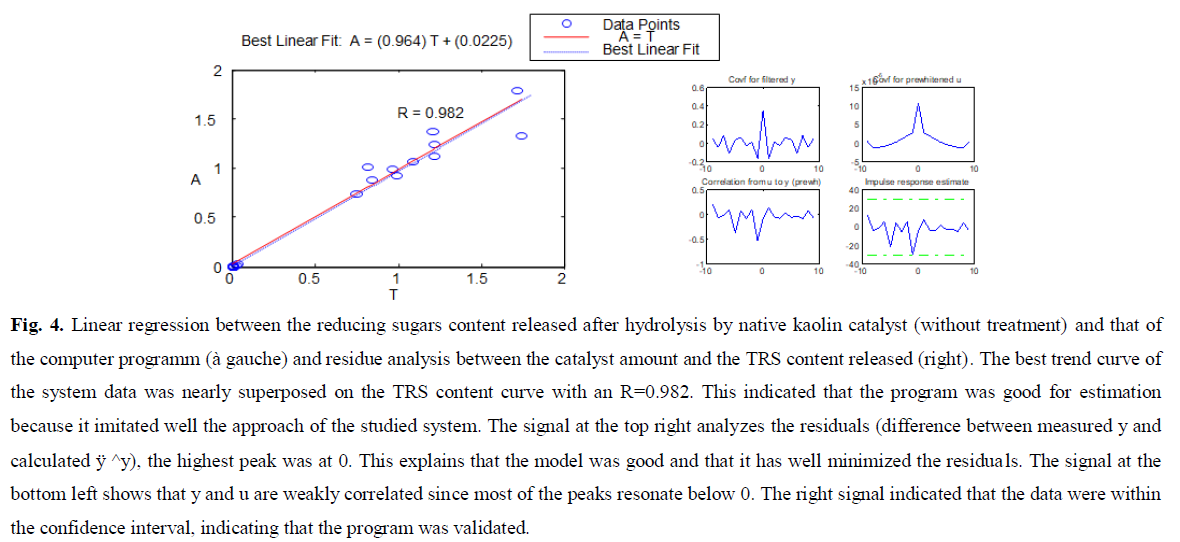
Fig 4: Linear regression between the reducing sugars content released after hydrolysis by native kaolin catalyst (without treatment) and that of the computer programm (à gauche) and residue analysis between the catalyst amount and the TRS content released (right). The best trend curve of the system data was nearly superposed on the TRS content curve with an R=0.982. This indicated that the program was good for estimation because it imitated well the approach of the studied system. The signal at the top right analyzes the residuals (difference between measured y and calculated ÿ ^y), the highest peak was at 0. This explains that the model was good and that it has well minimized the residuals. The signal at the bottom left shows that y and u are weakly correlated since most of the peaks resonate below 0. The right signal indicated that the data were within the confidence interval, indicating that the program was validated.
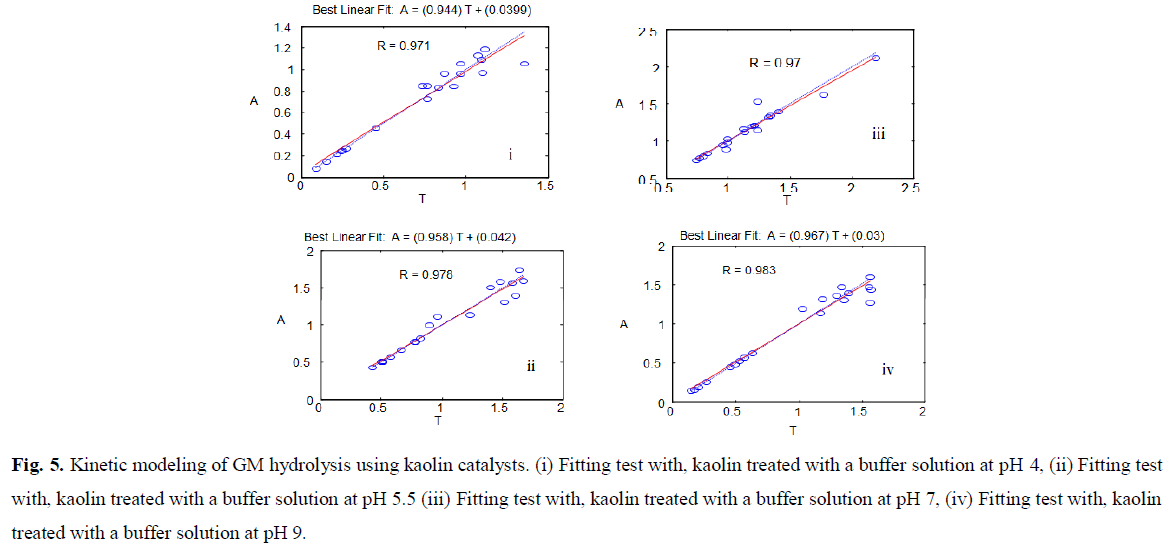
Fig 5: Kinetic modeling of GM hydrolysis using kaolin catalysts. (i) Fitting test with, kaolin treated with a buffer solution at pH 4, (ii) Fitting test with, kaolin treated with a buffer solution at pH 5.5 (iii) Fitting test with, kaolin treated with a buffer solution at pH 7, (iv) Fitting test with, kaolin treated with a buffer solution at pH 9.
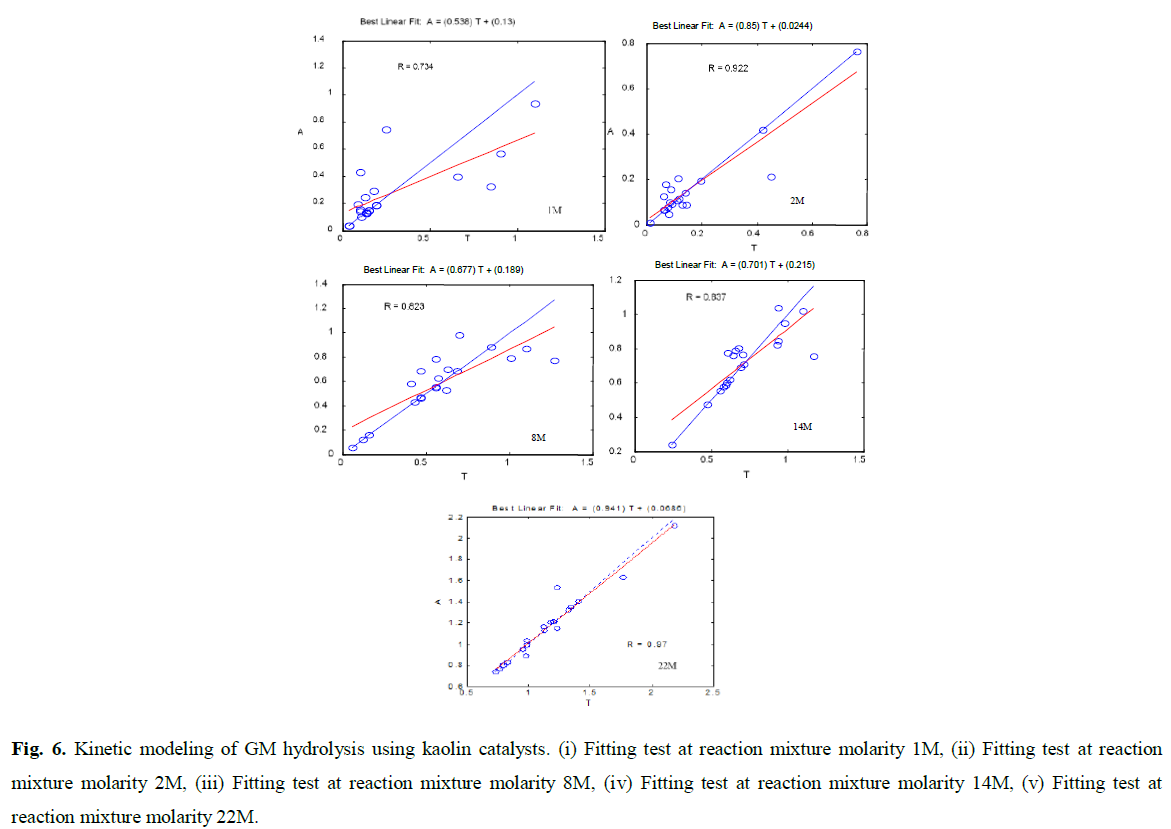
Fig 6: Kinetic modeling of GM hydrolysis using kaolin catalysts. (i) Fitting test at reaction mixture molarity 1M, (ii) Fitting test at reaction mixture molarity 2M, (iii) Fitting test at reaction mixture molarity 8M, (iv) Fitting test at reaction mixture molarity 14M, (v) Fitting test at reaction mixture molarity 22M.
Based on the fitting of the set of experimental data, we noticed that the model reproduced the evolution of the simple sugars content generated during the hydrolysis in most studied parameters.
All the other catalysts (pH=5.5, 7, and 9) showed the same aspect and validate the program (Fig.5).
For example, a native catalyst amount between 0.01 and 0.045 gives very satisfactory results. However, it was better to proceed with high catalyst amount in this kind of reaction. In fact, a zeolite of type H was used by (Moreau et al., 2000) to study the hydrolysis of sucrose. They demonstrated that the increase in the quantity of catalyst does not increase the rate of reaction but delays the appearance of 5-hydroxymethylfurfural (a toxic compound). 5-Hydroxymethylfurfural can be eliminated together with the catalyst in continuous operations. Several Studies reported efficient conversion of cellulose in the presence of an acidic monofunctional catalyst with high BrØnsted acidity (Onda et al., 2008; Shimizu et al., 2009; Suganuma et al., 2008).
Since the hydrolysis of polysaccharides such cellulose is directly correlated to the Brönsted acid sites, the natural clay mineral kaolinite might be an effective solid catalyst for the depolymerization of GM to reducing sugars (Yang et al., 2020). Certain modifications to this catalyst's chemical structure were performed to improve its performance. Experimental support was obtained for a ion-exchange model based on the principle of hard and soft acids and bases or HSAB principle (Xu and Harsh, 1990), applied to monovalent cation exchange on clay minerals, oxides, and exchange resins. This principle is as follows: Hard acids tend to be associated with hard bases and soft acids with soft bases. Assuming that kaolin catalyst was a soft acid, a treatment with formic acid allows us to describe:
Although this equation was considered unfavorable to a right shift, it allowed us to note some very useful information. The use of AlCl3 or ROH (as a hard base) requires adequate control and analysis methods. It was preferable to use compounds such as HCOOH, CH3COONa, and H3BO3, where we could predict the dissociation of these complexes or calculate the pH, at any stage of the reaction. According to the findings, treatment with pH solutions was determined to improve the reaction's conditions (Fig. 5).
The reducing sugar content increased at pH values of 4 and 7 and slightly decreased at pH values of 7 and 9. These variations dependent on pH matched the results of the computer program. In this study, it was found that the addition of formic acid to a reaction medium gave the following results:
The porous structure of the catalysts was also mentioned as a preponderant parameter in the conversion of cellulose. Indeed, the studies of Takagaki, and Van de Vyver, (2010) show that a mesoporous support (carbon nanofibers or a lamellar support (HNbMoO6) facilitated the polysaccharides accessibility within the catalysts, which increases their conversion (Takagaki et al., 2008). Comparison between experimental data and regression data: effect of formic acid concentration (1M, 2M, 8M, 14M and 22M) and catalyst amount were represented in the following fitting (Fig. 6).
From these results, we note that this approach does not improve the performance of the catalyst in a satisfactory way. Zaman et al., (2002), noted that kaolin has ends and faces. The ends contain silica and aluminum and the faces contain only silica (Zaman et al., 2002). The isoelectric pH of silica and aluminum have values between 2.0-3.5 and 8.5-10.4 respectively (Zaman and Freeman, 2003). On the other hand, Fripiat et al., (1971) showed that a treatment with 2N HCl allows the extraction of 15% of silica and 40% of aluminium (Ali et al., 2019; Al-Zahrani and Abdul-Majid, 2009; Fripiat et al., 1971). Some aspects of catalysis using kaolin are difficult to elucidate.
Conclusion
This paper describes our innovative, ecologically friendly chemical process for hydrolyzing galactomannan into galactose and mannose without significant raw material degradation. The heterogeneous catalytic conversion of GM is an ambitious process because of the inert nature of MG and the insufficient knowledge of solid-solid reaction systems. Our research using aluminosilicate kaolin H+ materials and reaction mixture acid/organic solvant demonstrated an ability to function as catalysts and catalytic support for GM conversion. The study's findings suggest that a variety of factors affect the reaction of O-glycosidic bond cleavage. These include temperature, catalyst concentration, solvent type, and pH. It appeared that the K4 kaolin catalyst better involved the breaking of the glycosidic bonds in galactomannan to release monomers.
Conflict of Interest
The authors declare no conflict of interest.
References
Albersheim, P., Nevins, D.J., English, P.D., Karr, A. (1967). A method for the analysis of sugars in plant cell-wall polysaccharides by gas-liquid chromatography. Carbohydrate Research, 5:340-345.
Ali, A.H., AL-Taie, M.H., Ayoob, I. (2019). The extraction of alumina from kaolin. Engineering and Technology Journal, 37:133-139.
Al-Zahrani, A.A. (2009). Extraction of alumina from local clays by hydrochloric acid process. Engineering Sciences, 20.
Amacher, M.C., Henderson, R.E., Breithaupt, M.D., Seale, C.L., LaBauve, J.M. (1990). Unbuffered and buffered salt methods for exchangeable cations and effective cation‐exchange capacity. Soil Science Society of America Journal, 54:1036-1042.
Audigie, C.I. (1978). Manipulations d'analyse biochimique.
Auxenfans, T., Buchoux, S., Djellab, K., Avondo, C., Husson, E., Sarazin, C. (2012). Mild pretreatment and enzymatic saccharification of cellulose with recycled ionic liquids towards one-batch process. Carbohydrate Polymers, 90:805-813.
Bouzouita, N., Khaldi, A., Zgoulli, S., Chebil, L., Chekki, R., Chaabouni, M.M., Thonart, P. (2007). The analysis of crude and purified locust bean gum: A comparison of samples from different carob tree populations in Tunisia. Food Chemistry, 101:1508-1515.
Dakia, P. (2009). Propriétés chimiques et rhéologiques des polysaccharides de l’endosperme des graines de caroubier (Ceratonia siliqua L.).
Dea, I.C., Clark, A.H., McCleary, B.V. (1986). Effect of galactose-substitution-patterns on the interaction properties of galactomannas. Carbohydrate Research, 147:275-294.
Dolcater, D.L., Lotse, E.G., Syers, J.K., Jackson, M.L. (1968). Cation exchange selectivity of some clay‐sized minerals and soil materials. Soil Science Society of America Journal, 32:795-798.
Fidan, H., Stankov, S., Petkova, N., Petkova, Z., Iliev, A., Stoyanova, M., Ercisli, S. (2020). Evaluation of chemical composition, antioxidant potential and functional properties of carob (Ceratonia siliqua L.) seeds. Journal of Food Science and Technology, 57:2404-2413.
Fripiat, J., Chaussidon, J., Jelli, A. (1971). Chimie-physique des phénomènes de surface: Applications aux oxydes et aux silicates. París: Masson, p:173.
Gillet, S. (2008). Etude des relations entre la Structure des Galactomannanes de Caroube et leurs Proprietes Fonctionnelles.
Huang, Y.B., Fu, Y. (2013). Hydrolysis of cellulose to glucose by solid acid catalysts. Green Chemistry, 15:1095-1111.
Ji, W., Shen, Z., Wen, Y. (2015). Hydrolysis of wheat straw by dilute sulfuric acid in a continuous mode. Chemical Engineering Journal, 260:20-27.
Google Scholar Crossref
Kassaye, S., Gupta, D., Pant, K.K., Jain, S. (2022). Valorization of microcrystalline cellulose using heterogeneous protonated zeolite catalyst: An experimental and kinetics approach. Reactions, 3:283-299.
Kassaye, S., Pagar, C., Pant, K.K., Jain, S., Gupta, R. (2016). Depolymerization of microcrystalline cellulose to value added chemicals using sulfate ion promoted zirconia catalyst. Bioresource Technology, 220:394-400.
Kosinov, N., Liu, C., Hensen, E.J., Pidko, E.A. (2018). Engineering of transition metal catalysts confined in zeolites. Chemistry of Materials, 30:3177-3198.
Kusema, B.T., Tönnov, T., Mäki-Arvela, P., Salmi, T., Willför, S., Holmbom, B., Murzin, D.Y. (2013). Acid hydrolysis of O-acetyl-galactoglucomannan. Catalysis Science and Technology, 3:116-122.
Linden, G., Lorient, D. (1994). Biochimie agro-industrielle : Valorisation alimentaire de la production agricole. Masson.
Liu, Y., Lei, F., He, L., Xu, W., Jiang, J. (2020). Comparative study on the monosaccharides of three typical galactomannans hydrolyzed by different methods. Industrial Crops and Products, 157:112895.
da Silva, J.L., Gonçalves, M.P. (1990). Studies on a purification method for locust bean gum by precipitation with isopropanol. Food Hydrocolloids, 4:277-287.
McCleary, B.V., Clark, A.H., Dea, I.C., Rees, D.A. (1985). The fine structures of carob and guar galactomannans. Carbohydrate Research, 139:237-260.
Moreau, C., Durand, R., Aliès, F., Cotillon, M., Frutz, T., Théoleyre, M.A. (2000). Hydrolysis of sucrose in the presence of H-form zeolites. Industrial Crops and Products, 11:237-242.
Nielsen, S.S. (2010). Food analysis (4th ed). Springer.
Onda, A., Ochi, T., Yanagisawa, K. (2008). Selective hydrolysis of cellulose into glucose over solid acid catalysts. Green Chemistry, 10:1033-1037.
Onda, A., Onda, S., Koike, M., Yanagisawa, K., Tsubaki, S., Hiraoka, M. (2017). Catalytic hydrolysis of polysaccharides derived from fast‐growing green macroalgae. Chem Cat Chem, 9:2638-2641.
Sakamoto, Y., Imamura, K., Onda, A. (2020). Hydrolysis of oligosaccharides and polysaccharides on sulfonated solid acid catalysts: Relations between adsorption properties and catalytic activities. ACS Omega, 5:24964-24972.
Shimizu, K.I., Furukawa, H., Kobayashi, N., Itaya, Y., Satsuma, A. (2009). Effects of Brønsted and Lewis acidities on activity and selectivity of heteropolyacid-based catalysts for hydrolysis of cellobiose and cellulose. Green Chemistry, 11:1627-1632.
Suganuma, S., Nakajima, K., Kitano, M., Yamaguchi, D., Kato, H., Hayashi, S., Hara, M. (2008). Hydrolysis of cellulose by amorphous carbon bearing SO3H, COOH, and OH groups. Journal of the American Chemical Society, 130:12787-12793.
Takagaki, A., Tagusagawa, C., Domen, K. (2008). Glucose production from saccharides using layered transition metal oxide and exfoliated nanosheets as a water-tolerant solid acid catalyst. Chemical Communications, 42:5363-5365.
Vilcocq, L., Castilho, P.C., Carvalheiro, F., Duarte, L.C. (2014). Hydrolysis of oligosaccharides over solid acid catalysts: A review. ChemSusChem, 7:1010-1019.
Xu, S., Harsh, J.B. (1990). Hard and soft acid‐base model verified for monovalent cation selectivity. Soil Science Society of America Journal, 54:1596-1601.
Yang, H., Tong, D., Dong, Y., Ren, L., Fang, K., Zhou, C., Yu, W. (2020). Kaolinite: A natural and stable catalyst for depolymerization of cellulose to reducing sugars in water. Applied Clay Science, 188:105512.
Zaman, A., Freeman, G. (2003). Methods for modifying high-shear rate properties of colloidal dispersions.
Zaman, A.A., Tsuchiya, R., Moudgil, B.M. (2002). Adsorption of a low-molecular-weight polyacrylic acid on silica, alumina, and kaolin. Journal of Colloid and Interface Science, 256:73-78.
Zhang, X., Zhang, Z., Wang, F., Wang, Y., Song, Q., Xu, J. (2013). Lignosulfonate-based heterogeneous sulfonic acid catalyst for hydrolyzing glycosidic bonds of polysaccharides. Journal of Molecular Catalysis A: Chemical, 377:102-107.
Author Info
S. Bouanani1,2* and A. Delimi2,32Department of Agronomy, Faculty of Natural and Life Sciences, University of Chadli Bendjedid El Tarf, Algeria
3Department of Biology, Faculty of Natural and Life Sciences, University of Chadli Bendjedid El Tarf, Algeria
Citation: Bouanani, S., Delimi, A. (2022). Valorization of galacomannan of carob seeds (Ceratonia siliqua) by total heterogenous hydrolysis with protonated-kaolin and computer program simulation. Ukrainian Journal of Ecology. 12: 28-38.
Received: 08-Nov-2022, Manuscript No. UJE-22-79308; , Pre QC No. P-79308; Editor assigned: 10-Nov-2022, Pre QC No. P-79308; Reviewed: 21-Nov-2022, QC No. Q-79308; Revised: 25-Nov-2022, Manuscript No. R-79308; Published: 30-Nov-2022, DOI: 10.15421/2022_411
Copyright: This is an open access article distributed under the terms of the Creative Commons Attribution License, which permits unrestricted use, distribution, and reproduction in any medium, provided the original work is properly cited.