Research - (2021) Volume 0, Issue 0
Technical, bioclimatic, and agro-technical foundations of revolutionary changes in sowing of field crops
V.Ya. Scherbakov1, O.O. Ozhovan1*, I.M. Kogut1 and A.O. Buyanovskiy2Abstract
During 40 years, the field and model experiments that showed the appreciable changes of technological parameters of seeding complex have been conducted. In particular, a significant change of sowing dates in the direction of later sowing of winter crops and extension of sowing period for spring crops, and even appearance of two optimal dates have been shown. From the point of view of sowing methods, the expediency of creation of square-shaped feeding area as an ideal configuration was disproved, and seeding rates for crops of continuous sowing method should preferably be reduced, relying on the compensator at the expense of individual productivity. It is also shown that it is advisable to sow seeds at a shallower depth than in the recommendations. All these changes are associated on the one hand with global warming, and on the other hand-with the introduction of modern innovative technologies.
Keywords
Sowing dates, sowing period, seeding rate, sowing depth, the configuration of the feeding area, strip-till technology, compensation by individual productivity, ladder morphology.
Introduction
The modern period of agrotechnological development is characterized by a comprehensive introduction of no-till systems into production. This trend did not emerge suddenly but resulted from a long and persistent study of the refusal of plowing. As early as 1899 published a book by I.E. Ovsinskij "New System of Farming", the author showed the effectiveness of shallow tillage and simultaneously proposed strip-tillage of wheat, which contradicted the even placement of plants in the area (Ovsinskij, 1899).
Half a century later, in the USA, I.E. Ovsinskij's ideas were supported by the agrarian E. Folkner (1959). In his work "The Madness of the Plowman," he convinces humankind, the plow for deep tillage is a disaster, even more than the company Krupp, which produces deadly weapons.
Many benefits in the development of no-till contributed to T.S. Maltsev (1954) and A.I. Baraev (1988), who developed a no-tillage system in Kazakhstan and Western Siberia.
In the conditions of the Ukrainian forest-steppe, the expediency of no-till was proved by F.T. Morgun (1981). This small review can be significantly expanded but given enough to understand that the idea of no-till has long been ripe and has under itself enough experimental evidence of expediency.
It is this hindsight that has given rise to today's innovative no-till, mini-till, and strip-till technologies. These technologies are based primarily on the performance of a set of technological operations for soil preparation, sowing, and fertilizer application in one pass of the machine. For transition to these innovative technologies, it is necessary to carry out complete technical re-equipment. Seeders, as such, will be replaced by the sowing combined unit, which is aggregated with tractors more potent than we had before. This technology significantly reduced fuel costs, achieved a high level of environmental friendliness, improved conditions for mineral fertilizers, slowed erosion, and, ultimately, increased yields.
Materials and Methods
Despite the high relevance of introducing innovative technologies, we will not discuss the technological consequences of introducing them. True, these consequences are caused by changing approaches to tillage and climatic factors, especially by significant warming (global warming). Let us consider this process without regard to global changes and the possibility of apocalyptic consequences of warming but only consider changes in some technological parameters. First, we should note the transition to later sowing of winter crops and earlier sowing of spring crops. Other indicators have also changed significantly: seeding rates, seed sowing depth, a width of row spacing. All this happened quickly and can be traced not as a slight difference but as significant differences, which are the subject of our research.
Our experiments are the result of painstaking observations over many years. The first unexpected results were obtained in experiments on the depth of sowing of grain sorghum seeds (1978-1983), then we were one of the first (1987-1989) to conclude the need to postpone the sowing of winter wheat to later dates. Experiments gave terrific results in 2017-2018, which showed the effectiveness of low seeding rates. In parallel, the effect of feeding area configuration on winter wheat production was traced. All these experiments were laid according to generally accepted methods, and the main results have been partially published in various scientific publications. However, our long-term work aims not to repeat previously published postulates but to use these materials to prove the need for radical changes in technological parameters. For example, we took only the sowing complex: 1) sowing period; 2) sowing method; 3) seeding rate; 4) seed sowing depth.
If we agree with our conclusions, it is necessary to carry out all further work on studying the issues of the sowing complex using those parameters that we presented as optimal.
Results
Let us consider all four elements of the sowing complex of winter wheat separately to ensure that significant changes are necessary. The transition from early to late sowing dates has been gradual, and it can be assumed that it is not completed to this day. If we take the last 50 years, during this period, the sowing dates became a whole month later (Table 1).
Years | |||||
---|---|---|---|---|---|
1970-1990 | 1990-2010 | 2010-2020 | |||
Optimal | Allowable | Optimal | Allowable | Optimal | Allowable |
Steppe | |||||
05.09-20.09 | 01.09-25.09 | 15.09-30.09 | 10.09-05.10 | 25.09-20.09 | 20.09-20.10 |
Forest Steppe | |||||
05.09-15.09 | 01.09-20.09 | 15.09-25.09 | 10.09-30.09 | 20.09-10.10 | 15.09-15.10 |
Polyssya | |||||
01.09-10.09 | 25.08-15.09 | 10.09-20.09 | 05.09-25.09 | 20.09-10.10 | 15.09-15.10 |
Table 1. Changes in optimal and permissible sowing dates of winter wheat.
Now there is an expansion of the zone of permissible dates, and we can expect that after a certain period, the whole of October will be defined as the permissible sowing date of winter wheat. In this regard, it seems appropriate for us to introduce instead of the term "sowing period" the new concept of "sowing period", especially since the optimal sowing dates come only in November for some varieties of double crops. Here the question arises: this shift in sowing flows is a consequence of global warming, or it is an integrated expression of the action of various factors. We believe that the cumulative effect of the factors that caused such a situation is the cumulative effect. In addition to warming, the constituent factors include the presence of varieties with a short stage of vernalization (30-35 days), lack of precursors, early release of the field, the need to avoid damage by cereal flies, and some diseases (WLCA), reduce weeds (winter storm "grasses Descurainia sophia, Capsella bursa-pastoris, Thlaspi arvense, which give seedlings in September). In addition, late sowing gives plants less clumping, which increases the illumination of the lower nodes and thus contributes to a better formation of sclerenchyma tissues, resulting in a higher level of plant resistance to lodging.
Winter barley now has a permissible sowing period from October 1 to November 1. Purely winter barley varieties should preferably be sown 5-7 days later than double-rowed barley.
Exciting changes have occurred regarding the sowing dates of winter rape. Here we are talking about the possibility of late dates and an increase in the sowing period from 10-12 to 40-45 days. Our research (Shherbakov et al., 2009) showed the expediency of sowing September 10-20 (Table 2).
Saw terms | Years | Average | ||
---|---|---|---|---|
1999 | 2000 | 2001 | ||
01.09 | 2,49 | 2,74 | 3,82 | 3,02 |
10.09 | 2,68 | 3,75 | 4,35 | 3,59 |
20.09 | 2,47 | 3,45 | 4,08 | 3,33 |
30.09 | 1,28 | 0,59 | 2,82 | 1,56 |
??? 0,5t | 0,19 | 0,22 | 0,23 | - |
Table 2. The yield of winter rape depending on sowing dates, t/ha.
As we can see, it is for sowing September 10-20 that higher and more stable yields were obtained. The lowest stability is characterized by late sowing, which in 2000 led to a crushing drop in productivity.
Today, producers choose the optimal sowing date, the period after precipitation in sufficient amount to obtain sprouts. Too early sowing has a consequence of overgrowth of plants, which necessitates the use of retardant. This circumstance has stretched the sowing period from August 10-15 to September 25-30. Producers use not only super-early but also super-penny sowing dates. Super-early sowing dates are not a deliberate decision of the producer but a consequence of the prolonged absence of precipitation (for example, sowing was done on September 15, but the sprouts were received only on October 15-20). This is not a reliable sowing date but often justifies expectations. There were many examples when they stopped the vegetation, formed only one true leaf, continued development in the spring, and eventually formed a yield of 2 t/ha more. Modern innovative technologies help improve seed germination conditions and mitigate the negative impact of violating the optimal sowing date.
In sunflower, the sowing period is almost the longest of all field crops. While the optimal sowing date for sunflowers in the southern Steppe is April 15-25, sunflowers are sown from April 5 to June 1. Such a range of dates is not a norm, but producers explain the advantages of sowing in late May or early June because the soil is better prepared for sowing and the field is free from weeds. In our observations in 2007, sunflower yields at optimum planting dates were lower than those of the extra-late June crop (Table 3).
Hybride | Saw terns | ||||
---|---|---|---|---|---|
10.04 | 25.04 | 10.05 | 25.05 | 10.06 | |
Dariy | 1,78 | 2,02 | 1,54 | 1,87 | 2,14 |
Zapovyte | 2,01 | 2,09 | 1,66 | 1,84 | 2,21 |
Table 3. Sunflower yield (tons/ha) depending on sowing dates in Kominternovsky District of Odessa Oblast, 2007.
We understand that this data cannot be regarded as probative scientific material because it is one year without repetition. However, if it still happens, this phenomenon cannot be ignored. Moreover, the conclusion arises that there is a lack of one-factor experiments with a widely deployed multivariate scheme in crop research in the last 30-40 years. If we compare classical parabolic dependence "factor parameter-yield" with the graphical model of one-factor multivariate experience, the graphical representation will be as follows (Fig. 1).
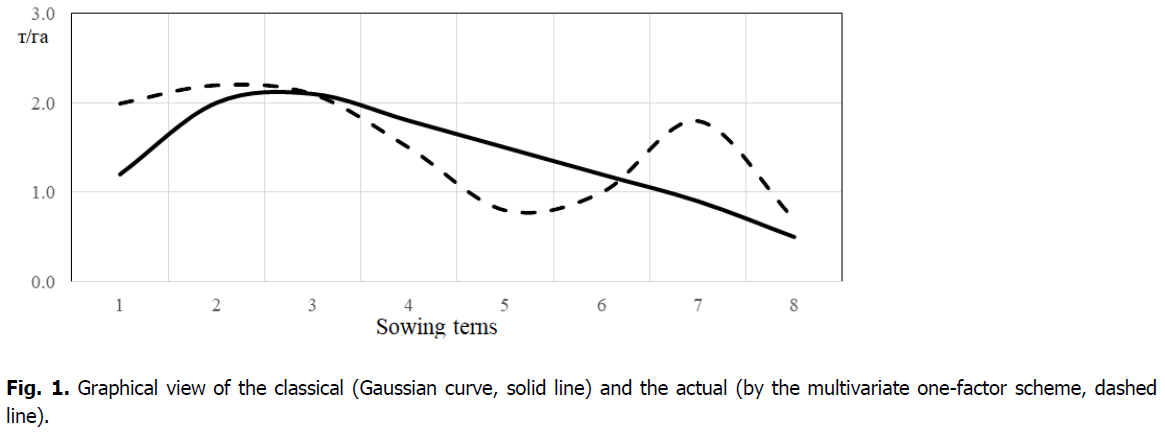
Figure 1: Graphical view of the classical (Gaussian curve, solid line) and the actual (by the multivariate one-factor scheme, dashed line).
The only correct way to find the optimal parameters of all technological measures is to set single-factor experiments with a wide range of options. Scientists have not noticed such a feature, so they mainly studied multifactorial schemes, where each of the factors has no more than 3-4 values. Let us consider everything that was noted earlier (new innovative technologies, climate change, fundamentally new varieties) and add our conclusion.
Changes in technological parameters affected the timing of sowing and other measures of the sowing complex. Let us consider whether it concerns methods of sowing, determining the configuration of the feeding area. Looking ahead, we want to note that this element, according to numerous observations, does not significantly impact plant productivity. For this reason, this element could be considered if not for one point, which is worth paying attention to. The generally accepted concept of the optimal configuration of the feeding area is considered a square, which provides a perfectly uniform placement of plants on the area.
Nevertheless, modern production practice has shown that this factor is by no means decisive for crop formation. For example, the best results are provided by sowing winter rape with 30-40 cm row spacing. Thus, on average for 2018-2019. The following yields were obtained in the farms of Berezovsky district of the Odessa region (Table 4).
Row width, cm | Plant number before the harvesting per 1 m2 | Yield, t/ha | Feeding area configuration, cmÃ?cm | |
---|---|---|---|---|
15 | 33 | 2,24 | 20 15 |
![]() |
23 | 34 | 2,38 | 23 13 |
![]() |
30 | 39 | 2,60 | 30 8,5 |
![]() |
40 | 38 | 2,74 | 40 6,6 |
![]() |
Table 4. The yield of winter rape depending on the width of the row spacing (At the rate of seeding 0.6 mln seeds per 1 ha).
Here it is vividly illustrated that a minimum yield is obtained by the configuration of the feeding area, which is close to the square. This example gives no reason to conclude that the feeding area distant from the square in configuration is the best. However, this fact allows us to conclude that the ideal configuration (square) is not necessary. It is this fact that prompted us to analyze the above dependence. One of the essential elements of the seeding complex, around which there is a constant discussion, is the seeding rate, which determines the density of the stems. It is well known that cereal grains can compensate for the insufficient number of plants by increasing clumpiness. Despite the presence of this compensator, most scientists tend to conclude that it is necessary to sow, for example, winter wheat with a seeding rate of 4.5-5.0 million ha of seeds. At the same time, Western Europe usually has a lower seeding rate-130-140 kg/ha (3.0-3.5 million seeds). To find optimal seeding rates under current no-till technologies, we researched 2017-2018, Modeling seeding rates from 1.5 to 4500000 seeds per 1 ha. The results were unexpected, as they did not confirm the view of a direct relationship between seeding rate and stem density (Table 5).
Seeding rate per 1 m2 | Number per 1 m2 | Stooling | Steems per 1 m2 | |||
---|---|---|---|---|---|---|
Seedlings | Full maturity plants | Total | Productive | Total | Productive | |
150 | 134 | 108 | 5,7 | 3,3 | 616 | 356 |
200 | 172 | 128 | 5,4 | 3,0 | 691 | 384 |
250 | 210 | 151 | 4,8 | 2,6 | 725 | 393 |
300 | 253 | 174 | 4,1 | 2,2 | 713 | 383 |
350 | 294 | 190 | 3,3 | 1,9 | 627 | 361 |
400 | 341 | 210 | 2,9 | 1,7 | 609 | 357 |
450 | 376 | 215 | 2,7 | 1,5 | 581 | 323 |
Table 5. Formation of stem density depending on seeding rate (average for 2017-2018).
Regarding the number of plants per unit area, the variants with the maximum seeding rate certainly had the advantage. However, the seeding rate of 2.0-3.0 million seeds per 1 ha was better by the number of generative organs. This divergence of conclusions can be explained by the fact that no-till technology significantly improves conditions for seed germination and further vegetative development of plants. In addition, when sowing at small rates, there is a marked increase in plant survival to the end of the growing season. This indicator gradually decreased in the transition from low to high seeding rates (Fig. 2).
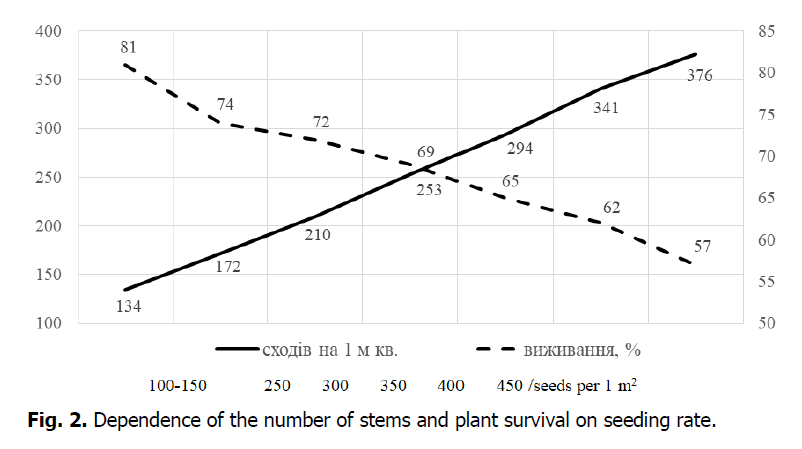
Figure 2: Dependence of the number of stems and plant survival on seeding rate.
If we consider plant activity in terms of efficiency concerning the productive number of stems to total, this indicator varied from 53,7 to 58,6%, and we were not able to identify any regularity of these changes. Schematically, the tillering of winter wheat at different seeding rates can be represented as follows (Fig. 3).
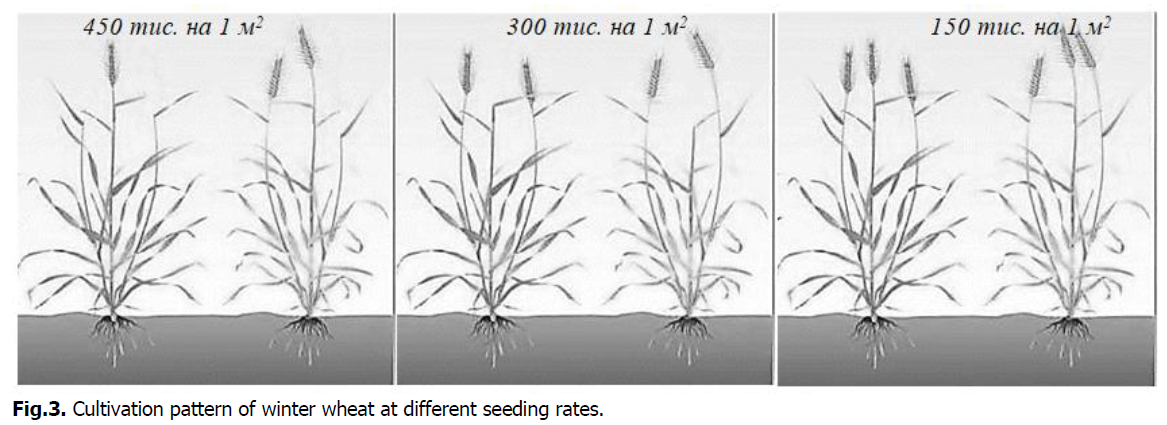
Figure 3: Cultivation pattern of winter wheat at different seeding rates.
Considering peculiarities of tillering and formation of the productive stem, the yield was reasonably expected (Table 6).
Saw rate, mln seeds/ha | Year | Average | Straw-seed ratio | |
---|---|---|---|---|
2017 | 2018 | |||
1,5 | 3,86 | 3,70 | 3,78 | 1,55 |
2,0 | 4,18 | 3,92 | 4,07 | 1,57 |
2,5 | 4,50 | 4,20 | 4,35 | 1,53 |
3,0 | 4,71 | 3,80 | 4,26 | 1,50 |
3,5 | 4,50 | 3,70 | 4,10 | 1,50 |
4,0 | 4,15 | 3,62 | 3,89 | 1,47 |
4,5 | 3,92 | 3,46 | 3,75 | 1,45 |
???05,? | 0,16 | 0,16 | - |
Table 6. The yield of winter wheat at different norms of seeding, t/ha.
As we can see, there is a steady advantage of seeding rates of 2.5-3.0 million seeds per 1 hectare. It was traced in both years, and the level of difference is significant and proven mathematically. Interestingly, the yield of straw is also dominated by low seeding rates, and the share of grain in the total biomass tends to grow in full for high seeding rates.
Winter rape can be considered a champion in reducing seeding rates over the past 25-30 years. The recommendations of 1990 suggested seeding 15 kg/ha of rape, which amounted to 5 million seeds per 1/ha. Already in 10 years, the seeding rate was reduced by a factor of 5, i.e., 1.0 million seeds per 1 ha (Novakovs'kij et al., 2000). In Germany in the 1980s, the seeding rate of rape was also limited to 1.0 million seeds per hectare (Sperber, 1987).
A little later, V.V. Lihochvor and V.F. Petrichenko (Petrichenko & Lihochvor, 2017) began to offer a seeding rate of 0.8 for varieties and 0.6-0.7 seeds/ha for hybrids. According to the recommendations of the relevant companies, many foreign hybrids are sown with a rate of 400 thousand seeds per 1 ha or 40 pcs/m2. It is believed that to form an expected yield, it is enough to have 20 rape plants per m2, which provides a sufficient yield at the expense of individual productivity. We checked the ability of winter rape due to the individual productivity of plants to compensate for thinness and came to a positive conclusion (Table 7).
Productivity elements | Plants per 1 m2 before the harvesting | |||||
---|---|---|---|---|---|---|
20 | 40 | 60 | 80 | 100 | ||
Number per plant | Flowers | 402 | 182 | 136 | 108 | 71 |
Pods | 292 | 145 | 102 | 71 | 50 | |
Seed number | per pod | 18 | 19 | 18 | 19 | 20 |
per plant | 5260 | 2750 | 1833 | 1355 | 1000 | |
Weight, g | 1000 seeds | 3,29 | 3,27 | 3,36 | 3,38 | 3,44 |
Sees per plant | 17,30 | 9,00 | 6,16 | 4,58 | 3,44 |
Table 7. Individual productivity of winter rape as a function of density (Model experience 2018).
Here we can trace due to which structural element the compensation occurs. First of all, it is the formation of more seeds on one plant, as such indices as the number of seeds in a pod and the weight of 1000 seeds vary in a relatively narrow range. This is quite understandable since the named indicators are genetically determined and vary only within the reaction norm. There is information in the scientific literature about the ability of winter rape to form 1500-1800 pods per plant. However, as a rule, in dense phytocenoses 1 plant forms 50-100 pods. Still, despite the astronomical compensatory possibilities of rapeseed, it is hardly justified to form sparse crops especially. We have at least two arguments for this conclusion:
Sparse plants have a too long generative period, which leads to the formation of pods in different conditions, and therefore the seeds have a large matriculate diversity. The first inflorescences are already overripe and fall off, and the last ones are still green, which makes harvesting difficult and increases losses;
On sparse crops, rape becomes less competitive against weeds, and thus, the crops become bogged down with a second generative wave. We noted concerning the two crops the tendency to significantly reduce seeding rates using no-till, mini-till, and strip-till-technologies. Nevertheless, we can give examples of the reverse order: for example, for corn in irrigation now recommend the density of 80-90 thousand/ha, and on land-45-55 thousand, while 20 years ago these figures were respectively 60-70 and 35-40 thousand/ha. Sunflower optimal density grew noticeably-55-60 thousand/ha against 35-40 thousand/ha before. We are not talking about the direction of change but about their presence and the need to consider when planning scientific research. At the beginning of our report, it was announced that under current conditions, when innovative technologies are widely implemented, changes in all parameters of sowing complex: terms and methods of sowing, seeding rates, and seed embedment depth are observed. Above were given the level and direction of these changes of the first three elements. The question of the optimum depth of seeding was not left unchanged. The fact is that the imperfect seeding technique and low quality of tillage mechanically inclined the general opinion to increase seeding depth. In retrospect, this figure looks as follows (Table 8).
Crops | Years | |||
---|---|---|---|---|
1960 | 1980 | 2000 | 2020 | |
Wheat, barley | 7-8 | 7-8 | 5-6 | 4-5 |
Corn | 10-12 | 8-10 | 7-8 | 6-7 |
Sorghum | 6-8 | 5-6 | 4-5 | 3-4 |
Peas | 8-9 | 7-8 | 7-8 | 5-6 |
Sunflower | 7-8 | 7-8 | 6-7 | 4-5 |
Winter rye rape | 5-6 | 4-5 | 3-4 | 3-4 |
Rice | 1,5-2,0 | 1,5-2,0 | 1,5-2,0 | 1,5-2,0 |
Table 8. Historical change in the depth of seeding of significant field crops.
This retrospective makes it possible to make sure that the reduction of the seed placement depth has a global nature because it concerns all crops. The only crop, the seeds of which are steadily earned in the soil to a 1.5-2.0 cm depth, is rice. Moreover, this is understandable: the soil in checks (cards) is perfectly aligned, and seeders are equipped with flaps, limiting the clump of the colter stroke. The conclusion is this: we do not sow deeply to meet the needs of the crop. However, because of uneven tillage, drying of the surface layer is more profound than the optimum of seeding, the inability of the seeder to provide a shallow and uniform depth.
The need to reduce the depth of seeding is an understandable phenomenon. Firstly, the nature of wild plants itself is by no means little adapted to the deep sinking of seeds into the ground. Secondly, a young seedling spends too much "effort" to cross a thick layer of soil and appear on the surface. More than 30 years ago, we concluded that shallow seeding has complex advantages over deep seeding, working with grain sorghum. The experiment was conducted on a perfectly aligned area and depth by pressing the seeds under the template. The results clearly illustrated the expediency of shallow seed embedding (Table 9).
Depth of sowing, cm | Duration of sowing-seedlings, days | First leaf characteristics | |||
---|---|---|---|---|---|
lenght, cm | width, cm | humidity, % | Chlorophile content, mg/g of dry matter | ||
1 | 16 | 3,1 | 0,82 | 77,3 | 3,8 |
2 | 13 | 3,3 | 0,76 | 79,0 | 3,7 |
3 | 11 | 3,4 | 0,73 | 79,4 | 3,5 |
4 | 14 | 3,6 | 0,69 | 79,9 | 3,3 |
5 | 15 | 3,9 | 0,64 | 80,3 | 3,0 |
6 | 17 | 3,9 | 0,60 | 84,1 | 3,1 |
7 | 18 | 4,1 | 0,56 | 85,5 | 2,9 |
8 | 19 | 4,3 | 0,51 | 86,0 | 2,7 |
Table 9. Characteristics of sorghum ladder obtained at different depths of seed embedding.
Of course, 1-2 cm is a depth beyond technical possibilities, and also at such a depth, all the sowing period (in the absence of precipitation) is dry. However, 3-4 cm is the depth, which provides the ladder with high content of dry matter and the main pigment of photosynthesis-chlorophyll. It should be noted that the difference in chlorophyll content between the extreme variants reaches 29%. This phenomenon gives a starting "head start" to those plants that emerged from seeds earned the lowest possible depth.
In 2019 we laid a model experiment with winter wheat. Moreover, here also showed the advantage of the ladder from the seed earned to a depth of 3 cm (Table 10).
Depth of sowing, cm | Duration of sowing-seedlings, days | First leaf hunidity, % | Tilling capacity | Kernels weight per spike, g | Yield, g/m2 |
---|---|---|---|---|---|
1 | 14 | 80,2 | 2,1 | 1,36 | 408 |
2 | 12 | 80,6 | 2,3 | 1,31 | 427 |
3 | 10 | 81,2 | 2,5 | 1,30 | 442 |
4 | 12 | 84,0 | 2,5 | 1,26 | 417 |
5 | 14 | 86,1 | 2,3 | 1,27 | 414 |
6 | 16 | 86,4 | 2,1 | 1,21 | 385 |
7 | 17 | 88,2 | 2,0 | 1,18 | 362 |
???05 | - | - | - | 0,08 | 28 |
Table 10. Peculiarities of winter wheat plants' growth and their productivity depending on the depth of seed sowing.
As can be seen, winter wheat seed has a similar response to the depth of embedding. The moisture content of the first leaf decreases by almost 8.0% in the ladder of seeds earned shallowly. Interestingly, plant productivity at shallow depth also increases markedly. Once again, we note: this was all obtained in model experiments, and therefore, the results are not a basis for recommendations for production. However, we also give them not for this reason but only to draw researchers' attention to the dissonance between the biological needs of culture and the actual values due to external factors.
Conclusions
Based on the analysis of many years of research carried out with different field crops and the study of global trends in technological parameters, the following conclusions can be made:
Modern innovative technologies have created prerequisites for changing technological parameters, in particular, sowing complex, namely:
The optimal sowing period should be considered as the optimal sowing period, in terms of duration, is much longer than previously defined dates; creation of the ideal configuration of the feeding area in the form of a square for the plants is not a prerequisite for the formation of maximum productivity; the seeding rate for most crops decreases markedly, but it grows both in dry and irrigated conditions for row crops.
The compensatory mechanism of crops of continuous sowing method is able not only to create stem density as in the case of high seeding rates but also to exceed it; the optimal sowing depth value gradually decreases, and the plants respond positively to this change, forming a resistance to adverse conditions stalks with increased photosynthetic energy due to the growth of chlorophyll content.
When developing research programs, it is necessary to consider the trends mentioned above and include in the schemes of experiments variants with values that significantly expand the spectrum of the factor. First of all, these should be single-factor experiments.
References
Baraev, ?.I. (1988). Pochvozashhitnoe zemledelie. Moscow. Agropromizdat.
Folkner, Je. (1959). Bezumija paharja: Gossel'hozzhz.
Mal'cev, T.S. (1954). Bezotval'naja obrabotka pochvy. Sverdlovsk.
Morgun, F.T. (1981). Pole bez pluga. Har'kov: Prapor.
Novakovs'kij, A.G., Kalashnik, V.?., Shherbakov, V.Ja., Zhuk ta?n., M.M. (2000). Rekomendac?? z tehnolog?? viroshhuvannja ta pererobki nas?nnja r?paka v Odes'k?j oblast?. Odesa: Astro Print.
Ovsinskij, I.E. (1899). Novaja sistema zemledelija. Kyiv: Typography of S.V. Kul'zhenko.
Petrichenko, V.F., Lihochvor, V.V. (2017). Suchasn? tehnolog?? u roslinnictv? v ?storichnomu rakurs? ?sv?tl? ?vro?ntegrac?jnih viklik?v. V?snik Agrarno? Nauki.
Shherbakova, V.Ja. (2009). Ozimij r?pak v Stepu Ukra?ni/[Shherbakov, V.Ja., Nereuc'kij S.G., Bodnar M.V. i dr.]; pod red. Odesa. Ltd ?NVAC.
Author Info
V.Ya. Scherbakov1, O.O. Ozhovan1*, I.M. Kogut1 and A.O. Buyanovskiy22Odesa Mechnikov National University, Odesa, Odessa Oblast, Ukraine
Citation: Scherbakov, V.Ya., Ozhovan, O.O., Kogut, I.M., Buyanovskiy, A.O. (2021). Technical, bioclimatic, and agro-technical foundations of revolutionary changes in sowing of field crops. Ukrainian Journal of Ecology, 11 (6), 69-76.
Received: 16-Jul-2021 Accepted: 29-Jul-2021 Published: 23-Aug-2021
Copyright: This is an open access article distributed under the terms of the Creative Commons Attribution License, which permits unrestricted use, distribution, and reproduction in any medium, provided the original work is properly cited.