Research - (2024) Volume 14, Issue 6
Origin of life is chemical synthesis and evolution, Cyanobacteria (Spirulina): Cellulose is a living cell
Man Gil Ahn*Abstract
Today, with the remarkable development of science, various chemical substances that are the origin of life are being discovered through space planetary exploration. The study of the origin of life is a very active field of research and important discoveries and progress are being made, but the mission of scientific research is to provide life, natural phenomena and evidence. There are various hypotheses about the origin of life on Earth since its birth 4.54 billion years ago. Space planets and asteroids, Membranes first: Lipid world, clay hypothesis, RNA world, Peptide hypothesis, Iron–sulfur world, Pre–cells (successive cellularisation), Deep sea hydrothermal vents, Life "seeded" from elsewhere, Carbonate-rich lakes. Panspermia, “A warm little pond": primordial soup, Miller–Urey experiment, PAH world hypothesis, Nucleobases and nucleotides, Geothermal springs. Nine requirements for the origin of Earth’s life: Not at the hydrothermal vent, but in a nuclear geyser system, (Maruyama, S., et al. 2019) A Nuclear Geyser Origin of Life Assembly Plant – Three-Step Model for the Emergence of the First Life on Earth and Cell Dynamics for the Coevolution of Life’s Functions (Maruyama, S., et al. 2023). At the bottom of the ocean, where light can't reach. It was discovered that methane, the substance of life, was leaking from seep under the seafloor in Chile (Schmidt Ocean Institute. 2024). We describe and encompass all hypotheses about the origin of life. Cyanobacteria are the most abundant taxonomic group to have ever existed on Earth and have continuously produced and released oxygen for billions of years, dramatically changing the composition of life on Earth from the anaerobic, weakly reducing pre-biotic atmosphere of early Earth to one of free gaseous oxygen.We present evidence based on the structural colors and life cycles of prokaryotes and eukaryotes. The cellulose of prokaryotes (cyanobacteria: Spirulina) and eukaryotes (marine macroalgae: green seaweed) is a living cell and a living organism. These cells commonly hibernate or are active in 1. dry environments, 2. aerobic, 3. anaerobic, 4. alkaline, 5. high and low temperatures. The cellulose of the cyanobacteria Spirulina contains chemically synthesized phycocyanin and as a protein, it has the chemical structure of a peptide. The phycocyanin pigment appeared as 1. blue to white, 2. white to bright-orange and 3. blue to bright-brown by photosynthetic reaction. Refraction of light allows photosynthetic organisms to store color, reflect and absorb light. As a phycocyanin pigment, blue is converted to white, light-orange and light-brown. The colors blue, white and brown appear green due to the refraction of light. As a phycocyanin pigment, protein peptides act as catalysts in photosynthetic reactions. When mixing Spirulina and blue pigments, blue is converted to green by photosynthetic reactions. As the origin of life on Earth, protein peptides of chemically synthesized phycocyanin pigments are alive in green as biochemical catalysts.
Spirulina phycocyanin pigment, a protein peptide, was a biochemically synthesized catalytic substance that gave birth to various organisms in the Primitive Earth ecological environment.
Keywords
Origin of life, Chemical synthesis and evolution, Prokaryotes, Cyanobacteria, Spirulina, Structural color, Life cycle, Phycocyanin, Peptides, Eukaryotes, Cellulose is alive.
Introduction
Spirulina Arthrospira is a prokaryotic plant of the Spirulinaceae, Cyanophyceae and Cyanobacteriota families and is a microalgae that is widely distributed worldwide. Spirulina is the dried biomass of blue-green algae (cyanobacteria) that can be consumed by humans and animals. The three species are Arthrospira platensis, A. fusiformis and A. maxima.Today, Spirulina is cultivated on land in various parts of the world, including North America, Africa, Europe and Asia and the dried product is packaged and sold as food. The drying temperature of Spirulina is 40-70°C and the drying method includes low-temperature drying and spray hot air drying. We are experiments were conducted by adding 0.01-0.2g of dried Spirulina to 1,000 cc of seawater or water and occasionally adding more water. When dried Spirulina is placed in seawater and water, the cells live and carry out vital activities through photosynthesis. (27-31 °C). The structural colors of Spirulina include green chlorophyll-a, b, blue, bright red (pink), orange, yellow, white, gray, brown and black. Based on environmental conditions, Spirulina lives by changing its structural colors into various colors under the influence of ultraviolet rays and temperature. The blue-green of Spirulina releases blue pigment through photosynthesis and the separated green is alive. The change in the structural colors of Spirulina is the signaling system of the chromosomes within the cells and changes to white, orange, yellow, brown, etc. and returns to green again. Spirulina produces a foul odor under anaerobic conditions and changes from green to black and when aerobic conditions occur, it begins to carry out vital activities with the cell's resilience. They change from green to white-gray-brown-black and then back to green. Spirulina is alive at 85-90 °C.
(Green-white, brown-green or green-green) Spirulina is alive at pH 3.5-3.7 and appears white or brown and then back to green. Dried Spirulina at 75-80 °C and then put it in water, it will change to white or brown and then back to green. The average period for Spirulina to change to various colors and back to green is about 10-20 days. The life cycle of Spirulina is constantly reproducing offspring through generational changes. Spirulina is a living organism composed of carbohydrates, proteins, lipids, nucleic acids and minerals and cellulose is a living organism. We have observed various changes in the ecological environment through structural colors and life cycles from Spirulina.
Structure and phycocyanin pigment of the cyanobacteria Spirulina
Information about the chemical structure and formula of phycocyanin:Structure: Phycocyanin is a monomer made up of an alpha (α) and beta (β) polypeptide. The α subunit is connected to a phycocyanobilin and the β subunit is connected to two phycocyanobilins through covalent bonds. Formula: The molecular formula for phycocyanin is C13H11N2O. Phycocyanin is a phycobiliprotein, which is a protein complex that absorbs and transfers specific wavelengths of light. Phycobiliproteins are part of phycobilisomes, which are large light harvesting antennae protein complexes. The shape of phycobilisomes can vary, but in species that lack phycoerythrin, they usually have at least two disks of phycocyanin per rod. Phycocyanin has many practical applications, including in food, cosmetics, pharmaceuticals and biomedical industries. It has antioxidant, anti-inflammatory and anti-aging properties (Fig. 1).
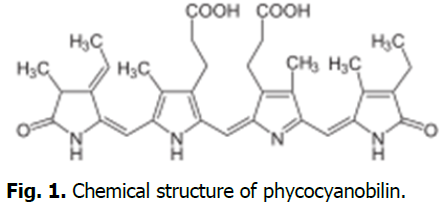
Fig. 1. Chemical structure of phycocyanobilin.
Phycocyanin separation from Spirulina
Phycocyanin is a type of blue pigment protein contained in algae (cyanobacteria (blue-green algae), red algae, cryptoalgae), marine macroalgae: green seaweed. (Ahn MG. 2021).The structural colors of phycocyanin pigments are 1. Blue to white. 2. White to light-orange. 3. Blue to light-brown. Biochemically synthesized phycocyanin pigments store, reflect and absorb color through the refraction of light (Fig. 2).
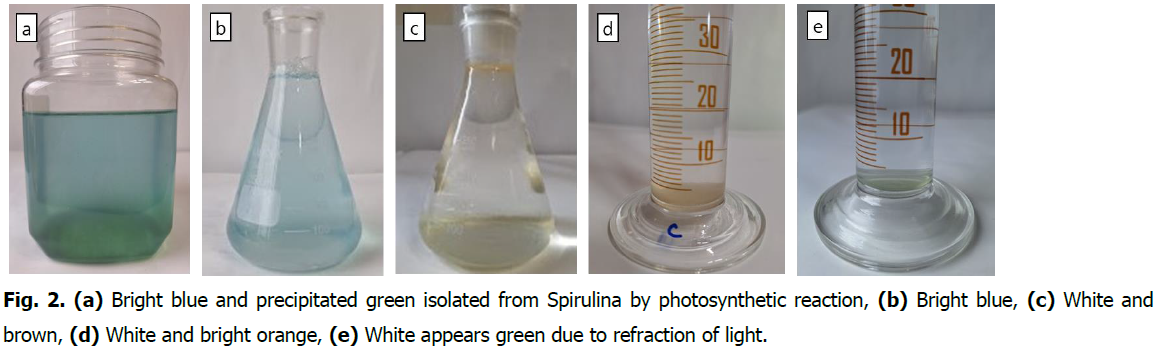
Fig. 2. (a) Bright blue and precipitated green isolated from Spirulina by photosynthetic reaction, (b) Bright blue, (c) White and brown, (d) White and bright orange, (e) White appears green due to refraction of light.
After separation of phycocyanin, the green is living
The green appears as white and brown through photosynthesis and then returns to green again (Fig. 3).
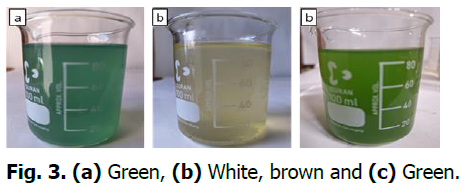
Fig. 3. (a) Green, (b) White, brown and (c) Green.
When Spirulina is mixed with picocyanin pigment, it transformed green (Fig. 4)
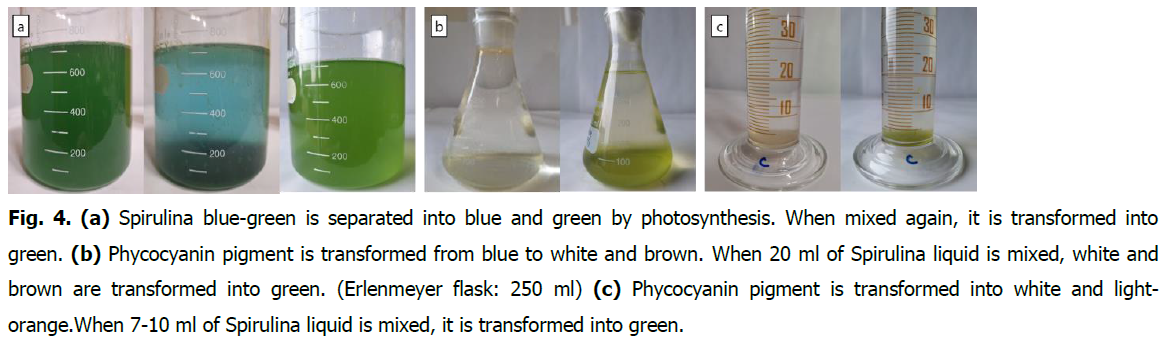
Fig. 4. (a) Spirulina blue-green is separated into blue and green by photosynthesis. When mixed again, it is transformed into green. (b) Phycocyanin pigment is transformed from blue to white and brown. When 20 ml of Spirulina liquid is mixed, white and brown are transformed into green. (Erlenmeyer flask: 250 ml) (c) Phycocyanin pigment is transformed into white and light-orange.When 7-10 ml of Spirulina liquid is mixed, it is transformed into green.
What is the feature of cyanobacteria Spirulina?
The structural colors of Spirulina transforming physiologically and perform vital activities under the influence of ultraviolet rays and temperature.1. Green to blue-green, 2. Green to bright red (pink), 3. Green to orange, 4. Green to yellow, 5. Green to white,6. Green to brown, 7. Green to white, 8. Green to gray, brown, black and back to green. 9. The bright red (pink) color of Spirulina appears when the cells are colonized. 10. The blue-green of dried Spirulina sometimes appears white when placed in seawater or water and then turns green again (Fig. 5).
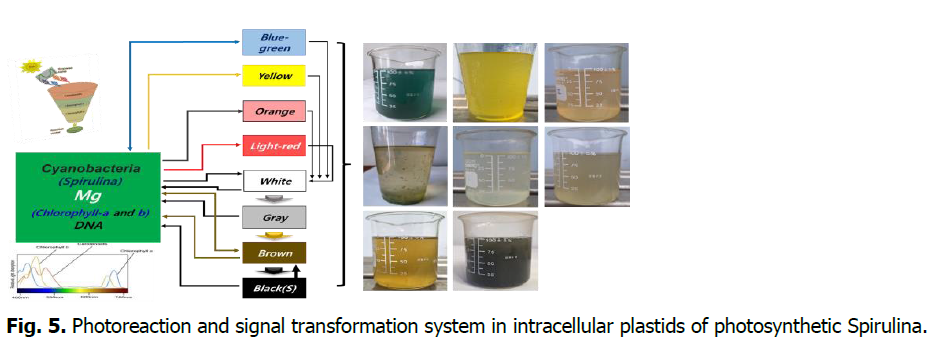
Fig. 5. Photoreaction and signal transformation system in intracellular plastids of photosynthetic Spirulina.
Materials and Methods
We applied dried Spirulina as a food product certified as organic in the United States. USDA: Spirulina: Powder Certified Organic Herbal Supplement. Various blue green algae. www.frontiercoop.com. 2020 Frontier Co-op. Norway, IA 52318 USA . 800-669-3275. S618888. Spirulina is a food product and the drying temperature is generally 40-70 degrees. The dried powder is a product dried by hot air. Spirulina is a biomass of cyanobacteria that can be consumed by humans and animals. There are three species: Arthrospira platensis, A. fusiformis, A. maxima (Fig. 6).
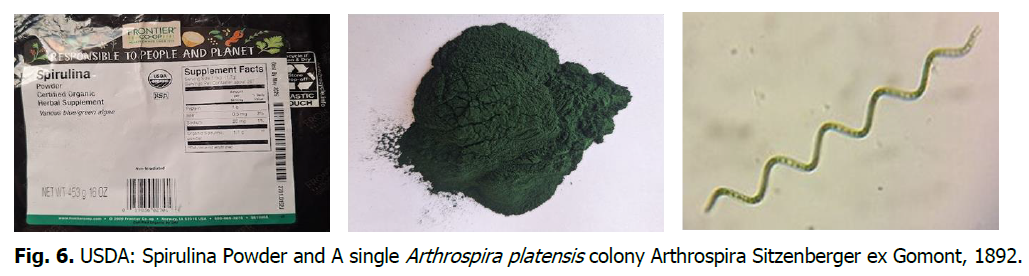
Fig. 6. USDA: Spirulina Powder and A single Arthrospira platensis colony Arthrospira Sitzenberger ex Gomont, 1892.
Results and Discussion
Dried Spirulina is alive
Dried Spirulina appears blue-green when placed in seawater or freshwater and is alive. When Spirulina is placed in seawater or freshwater, dormant cells slowly become active and then become very active at a temperature of 30-31 °C. Spirulina transforms from green to white and then turns green again and is alive (Fig. 7).
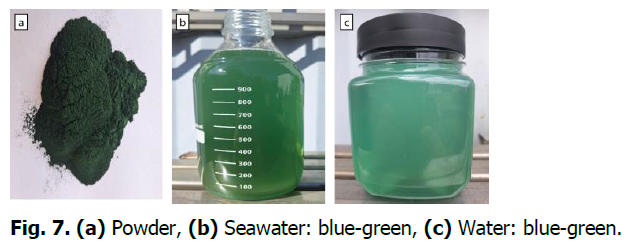
Fig. 7. (a) Powder, (b) Seawater: blue-green, (c) Water: blue-green.
Spirulina is alive at 85-90 °C
After adding Spirulina to water and stirring, 80 °C water and 100 °C water were mixed and it appeared from green to white and then back to green again. The average temperature of the water tested was 85-90 °C (Fig. 8).
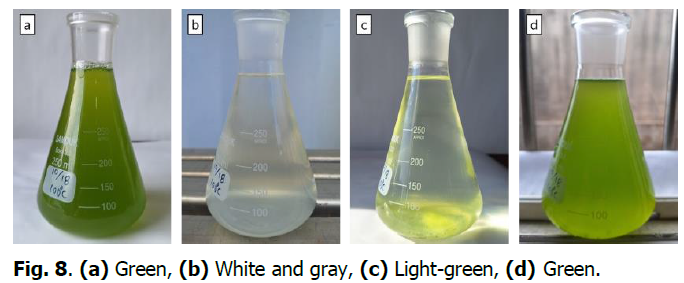
Fig. 8. (a) Green, (b) White and gray, (c) Light-green, (d)Green.
The cellulose in dried Spirulina is living cells
Spirulina was de-greened with a sulfuric acid solution at pH 3.5-3.7. It appeared white and brown and then turned green again. The drying temperature of Spirulina is 75-80°C and the Spirulina that appeared from green to white or brown changed back to green (Fig. 9).
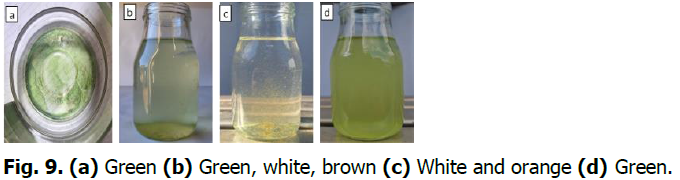
Fig. 9. (a) Green (b) Green, white, brown (c) White and orange (d)Green.
Spirulina living in anaerobic conditions
It can also occur during the process of greenery becoming polluted and emit a foul odor. When under anaerobic conditions, it appears from green to white, gray, brown and black.When under aerobic conditions, it appears again as green (Fig. 10).
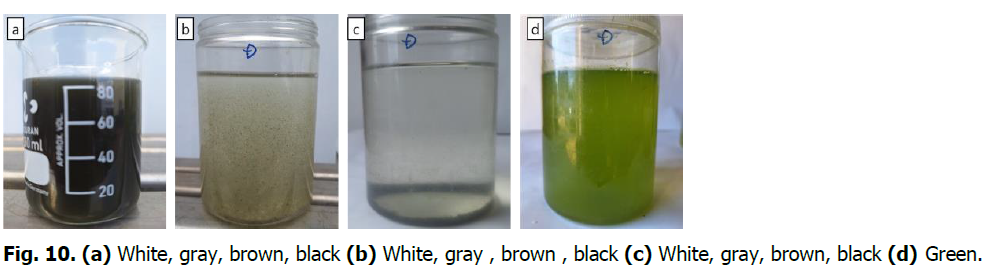
Fig. 10. (a) White, gray, brown, black (b) White, gray , brown , black (c) White, gray, brown, black (d)Green.
Structural colors and pigments of photosynthetic eukaryotic green seaweeds
The color of the cells is determined through a signal transmission system by nine types of genetic information in the mitochondria of green seaweed. (Ahn MG. 2021). The process chromaticity transformation of green seaweed.
- Green-Blue-Yellow-White-Pink (Orange)-Red–red(Purple)-Brown-Black-Green
- Green-white-pink-red-purple-brown-black-Green
- Green-white-pink-red-purple-brown-Green
- Green-white-pink–red (purple)-Green
- Green-white-pink-red-Green
- Green-white-pink-Green
- Green-white-Green
- Green seaweed is sometimes changing in chromatic depending on environmental conditions. (Green-brown-Green, Green-brown-white-Green, Green-blue-Green)
- This is when green seaweed is in the upper layer of seawater. Green-yellow-white-Green. Green-yellow-Green
- Marine macroalgae: Nine color of Green seaweed (Fig. 11).
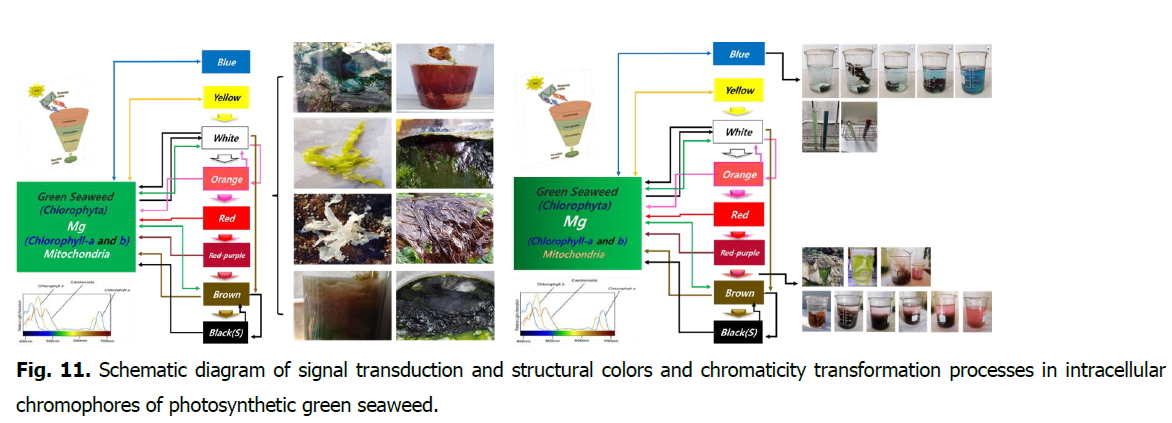
Fig. 11. Schematic diagram of signal transduction and structural colors and chromaticity transformation processes in intracellular chromophores of photosynthetic green seaweed.
Conclusion
There are various materials and hypotheses about the origin of life. The discovery and evidence of new organisms can further advance the understanding of the origin of life.
Acknowledgement
None.
Conflict of Interest
The authors declare no conflict of interest.
References
Maruyama, S., Ebisuzaki, T. (2023). A nuclear geyser origin of life: Life assembly plant–three‐step model for the emergence of the first life on earth and cell dynamics for the coevolution of life's functions. Conflicting Models for the Origin of Life 327-359.
Google Scholar, Crossref, Indexed at
Ahn, M. G. (2021). “The origin of life is chemical synthesis” marine algae (green seaweed): Cellulose is a cell. Ukrainian Journal of Ecology 11:55-67.
Ahn, M. G. (2021). Separation and method of natural pigments by chromaticity transformation of green seaweed. Ukrainian Journal of Ecology 11:37-46.
Papastavrou, N., Horning, D. P., Joyce, G. F. (2024). RNA-catalyzed evolution of catalytic RNA. Proceedings of the National Academy of Sciences 121:e2321592121.
Google Scholar, Crossref, Indexed at
Mann, A. (2021). Making headway with the mysteries of life’s origins. Proceedings of the National Academy of Sciences, 118:e2105383118.
Google Scholar, Crossref, Indexed at
Zurich, E. T. H. (2020). Uncovering mysteries of earth’s primeval atmosphere 4.5 billion years ago and the emergence of life.
Y. Furukawa, Y., Chikaraishi, Y., Ohkouchi, N., Ogawa, N. O., Glavin, D. P., Dworkin, J. P., Nakamura, T. (2019). Extraterrestrial ribose and other sugars in primitive meteorites. Proceedings of the national academy of sciences 116:24440-24445.
Google Scholar, Crossref, Indexed at
Maruyama, S., Kurokawa, K., Ebisuzaki, T., Sawaki, Y., Suda, K., Santosh, M. (2019). Nine requirements for the origin of Earth's life: Not at the hydrothermal vent, but in a nuclear geyser system. Geoscience Frontiers 10:1337-1357.
Google Scholar, Crossref, Indexed at
Zimmer, C. (2017). Scientists say Canadian bacteria fossils may be earth's oldest. The New York Times 2.
Scharf, C., Virgo, N., Cleaves, H. J., Aono, M., Aubert-Kato, N., Aydinoglu, A., Yabuta, H. (2015). A strategy for origins of life research.
Google Scholar, Crossref, Indexed at
Dodd, M. S., Papineau, D., Grenne, T., Slack, J. F., Rittner, M., Pirajno, F., Little, C. T. (2017). Evidence for early life in Earth’s oldest hydrothermal vent precipitates. Nature 543:60-64.
Google Scholar, Crossref, Indexed at
Peretó, J. (2005). Controversies on the origin of life. International Microbiology 8:23–31.
Warmflash, D., Weiss, B. (2005). Did life come from another world?. Scientific American 293:64-71.
Google Scholar, Crossref, Indexed at
NASA. (2015). NASA cooks up icy organics to mimic life's origins.
Miller, S. L. (1953). A production of amino acids under possible primitive earth conditions. Science 117:528-529.
Google Scholar, Crossref, Indexed at
Glazer, A. N. (1989). Light guides: Directional energy transfer in a photosynthetic antenna. Journal of Biological Chemistry 264:1-4.
Google Scholar, Crossref, Indexed at
Ratha, S. K., Prasanna, R. (2012). Bioprospecting microalgae as potential sources of “Green Energy”—challenges and perspectives. Applied Biochemistry and Microbiology 48:109-125.
Brient, L., Lengronne, M., Bertrand, E., Rolland, D., Sipel, A., Steinmann, D., Bormans, M. (2008). A phycocyanin probe as a tool for monitoring cyanobacteria in freshwater bodies. Journal of Environmental Monitoring, 10:248-255.
Google Scholar, Crossref, Indexed at
Wang, X. Q., Li, L. N., Chang, W. R., Zhang, J. P., Gui, L. L., Guo, B. J., Liang, D. C. (2001). Structure of C-phycocyanin from Spirulina platensis at 2.2 Å resolution: A novel monoclinic crystal form for phycobiliproteins in phycobilisomes. Acta Crystallographica Section D: Biological Crystallography 57:784-792.
Google Scholar, Crossref, Indexed at
Adir, N., Vainer, R., Lerner, N. (2002). Refined structure of C-phycocyanin from the cyanobacterium Synechococcus vulcanus at 1.6 Å: Insights into the role of solvent molecules in thermal stability and co-factor structure. Biochimica et Biophysica Acta (BBA)-Bioenergetics 1556:168-174.
Google Scholar, Crossref, Indexed at
Ong, L. J., Glazer, A. N. (1987). R-phycocyanin II, a new phycocyanin occurring in marine Synechococcus species. Identification of the terminal energy acceptor bilin in phycocyanins. Journal of Biological Chemistry 262:6323-6327.
Google Scholar, Crossref, Indexed at
Pizarro, S. A., Sauer, K. (2001). Spectroscopic study of the light‐harvesting protein C‐phycocyanin associated with colorless linker peptides. Photochemistry and Photobiology 73:556-563.
Google Scholar, Crossref, Indexed at
Glazer, A. N., Fang, S., Brown, D. M. (1973). Spectroscopic properties of C-phycocyanin and of its α and β subunits. Journal of Biological Chemistry 248:5679-5685.
Google Scholar, Crossref, Indexed at
Stanier, R. Y., Kunisawa, R., Mandel, M. C. B. G., Cohen-Bazire, G. (1971). Purification and properties of unicellular blue-green algae (order Chroococcales). Bacteriological Reviews 35:171-205.
Google Scholar, Crossref, Indexed at
Buchweitz, M. (2024). Natural solutions for blue colors in food. Woodhead Publishing 437-464.
Google Scholar, Crossref, Indexed at
Woźniak, M., Bradtke, K. M., Darecki, M., Krężel, A. (2016). Empirical model for phycocyanin concentration estimation as an indicator of cyanobacterial bloom in the optically complex coastal waters of the Baltic Sea. Remote Sensing 8:212.
Google Scholar, Crossref, Indexed at
Kuddus, M., Singh, P., Thomas, G., Al-Hazimi, A. (2013). Recent developments in production and biotechnological applications of C‐phycocyanin. BioMed Research International 2013:742859.
Google Scholar, Crossref, Indexed at
Marquez, F. J., Sasaki, K., Kakizono, T., Nishio, N., Nagai, S. (1993). Growth characteristics of Spirulina platensis in mixotrophic and heterotrophic conditions. Journal of Fermentation and Bioengineering 76:408-410.
Google Scholar, Crossref, Indexed at
Ortiz-Álvarez, R., de Los Ríos, A., Fernández-Mendoza, F., Torralba-Burrial, A., Pérez-Ortega, S. (2015). Ecological specialization of two photobiont-specific maritime cyanolichen species of the genus Lichina. PLoS One 10:e0132718.
Google Scholar, Crossref, Indexed at
Liu, J., Zhang, X., Sui, Z., Zhang, X., Mao, Y. (2005). Cloning and characterization of C-phycocyanin operon from the cyanobacterium Arthrospira platensis FACHB341. Journal of Applied Phycology 17:181-185.
Google Scholar, Crossref, Indexed at
Guan, X., Qin, S., Su, Z., Zhao, F., Ge, B., Li, F., Tang, X. (2007). Combinational biosynthesis of a fluorescent cyanobacterial holo-α-phycocyanin in Escherichia coli by using one expression vector. Applied Biochemistry and Biotechnology 142:52-59.
Google Scholar, Crossref, Indexed at
Ohta, N., Matsuzaki, M., Misumi, O., Miyagishima, S. Y., Nozaki, H., Tanaka, K., Kuroiwa, T. (2003). Complete sequence and analysis of the plastid genome of the unicellular red alga Cyanidioschyzon merolae. DNA Research, 10:67-77.
Google Scholar, Crossref, Indexed at
Tooley, A. J., Cai, Y. A., Glazer, A. N. (2001). Biosynthesis of a fluorescent cyanobacterial C-phycocyanin holo-α subunit in a heterologous host. Proceedings of the National Academy of Sciences 98:10560-10565.
Google Scholar, Crossref, Indexed at
Eriksen, N. T. (2008). Production of phycocyanin-a pigment with applications in biology, biotechnology, foods and medicine. Applied Microbiology and Biotechnology 80:1-14.
Google Scholar, Crossref, Indexed at
Guo, N., Zhang, X., Lu, Y., Song, X. (2007). Analysis on the factors affecting start-up intensity in the upstream sequence of phycocyanin β subunit gene from Arthrospira platensis by site-directed mutagenesis. Biotechnology Letters 29459-464.
Google Scholar, Crossref, Indexed at
Lu, Y., Zhang, X. (2005). The upstream sequence of the phycocyanin β subunit gene from Arthrospira platensis regulates expression of gfp gene in response to light intensity. Electronic Journal of Biotechnology 8:0-0.
Google Scholar, Crossref, Indexed at
Sloth, J. K., Wiebe, M. G., Eriksen, N. T. (2006). Accumulation of phycocyanin in heterotrophic and mixotrophic cultures of the acidophilic red alga Galdieria sulphuraria. Enzyme and Microbial Technology 38:168-175.
Google Scholar, Crossref, Indexed at
Chaneva, G., Furnadzhieva, S., Minkova, K., Lukavsky, J. (2007). Effect of light and temperature on the cyanobacterium Arthronema africanum-a prospective phycobiliprotein-producing strain. Journal of Applied Phycology 19:537-544.
Google Scholar, Crossref, Indexed at
Venugopal, V., Prasanna, R., Sood, A., Jaiswal, P., Kaushik, B. D. (2006). Stimulation of pigment accumulation in Anabaena azollae strains: Effect of light intensity and sugars. Folia Microbiologica 51:50-56.
Google Scholar, Crossref, Indexed at
Narayan, M. S., Manoj, G. P., Vatchravelu, K., Bhagyalakshmi, N., Mahadevaswamy, M. (2005). Utilization of glycerol as carbon source on the growth, pigment and lipid production in Spirulina platensis. International Journal of Food Sciences and Nutrition 56:521-528.
Google Scholar, Crossref, Indexed at
Troxler, R. F., Ehrhardt, M. M., Brown-Mason, A. S., Offner, G. D. (1981). Primary structure of phycocyanin from the unicellular rhodophyte Cyanidium caldarium. II. Complete amino acid sequence of the beta subunit. Journal of Biological Chemistry 256:12176-12184.
Google Scholar, Crossref, Indexed at
Romay, C. H., Armesto, J., Remirez, D., González, R., Ledon, N., Garcia, I. (1998). Antioxidant and anti-inflammatory properties of C-phycocyanin from blue-green algae. Inflammation Research 47:36-41.
Google Scholar, Crossref, Indexed at
Romay, C. H., Gonzalez, R., Ledon, N., Remirez, D., Rimbau, V. (2003). C-phycocyanin: A biliprotein with antioxidant, anti-inflammatory and neuroprotective effects. Current Protein and Peptide Science 4:207-216.
Google Scholar, Crossref, Indexed at
Romay, C., Ledón, N., González, R. (1998). Further studies on anti-inflammatory activity of phycocyanin in some animal models of inflammation. Inflammation Research 47:334-338.
Google Scholar, Crossref, Indexed at
Patel, A., Mishra, S., Ghosh, P. K. (2006). Antioxidant potential of C-phycocyanin isolated from cyanobacterial species Lyngbya, Phormidium and Spirulina spp.
Zhou, Z. P., Liu, L. N., Chen, X. L., Wang, J. X., chen, M. I. N., Zhang, Y. Z., Zhou, B. C. (2005). Factors that effect antioxidant activity of C‐phycocyanins from Spirulina platensis. Journal of Food Biochemistry 29:313-322.
Min, S. K., Park, J. S., Luo, L., Kwon, Y. S., Lee, H. C., Jung Shim, H., Shin, H. S. (2015). Assessment of C-phycocyanin effect on astrocytes-mediated neuroprotection against oxidative brain injury using 2D and 3D astrocyte tissue model. Scientific Reports 5:14418.
Google Scholar, Crossref, Indexed at
Liu, Q., Huang, Y., Zhang, R., Cai, T., Cai, Y. (2016). Medical application of Spirulina platensis derived C‐phycocyanin. Evidence‐Based Complementary and Alternative Medicine 2016:7803846.
Google Scholar, Crossref, Indexed at
Vadiraja, B. B., Gaikwad, N. W., Madyastha, K. M. (1998). Hepatoprotective effect of C-Phycocyanin: Protection for carbon tetrachloride and R-(+)-pulegone-mediated hepatotoxicty in rats. Biochemical and Biophysical Research Communications 249:428-431.
Google Scholar, Crossref, Indexed at
Basha, O. M., Hafez, R. A., El-Ayouty, Y. M., Mahrous, K. F., Bareedy, M. H., Salama, A. M. (2008). C-Phycocyanin inhibits cell proliferation and may induce apoptosis in human HepG2 cells. Egypt J Immunol 15:161-167.
Liu, Q., Huang, Y., Zhang, R., Cai, T., Cai, Y. (2016). Medical application of Spirulina platensis derived C‐phycocyanin. Evidence‐Based Complementary and Alternative Medicine 2016:7803846.
Google Scholar, Crossref, Indexed at
Pardhasaradhi, B. V., Ali, A. M., Kumari, A. L., Reddanna, P., Khar, A. (2003). Phycocyanin-mediated apoptosis in AK-5 tumor cells involves down-regulation of Bcl-2 and generation of ROS. Molecular Cancer Therapeutics 2:1165-1170.
Martelli, G., Folli, C., Visai, L., Daglia, M., Ferrari, D. (2014). Thermal stability improvement of blue colorant C-Phycocyanin from Spirulina platensis for food industry applications. Process Biochemistry 49:154-159.
Google Scholar, Crossref, Indexed at
Chaiklahan, R., Chirasuwan, N., Bunnag, B. (2012). Stability of phycocyanin extracted from Spirulina sp.: Influence of temperature, pH and preservatives. Process Biochemistry 47:659-664.
Google Scholar, Crossref, Indexed at
Author Info
Man Gil Ahn*Citation: Ahn, MG. (2024). Origin of life is chemical synthesis and evolution, Cyanobacteria (Spirulina): Cellulose is a living cell. Ukrainian Journal of Ecology. 14:1-8.
Received: 02-Nov-2024, Manuscript No. UJE-24-153036; , Pre QC No. P-153036; Editor assigned: 04-Nov-2024, Pre QC No. P-153036; Reviewed: 18-Nov-2024, QC No. Q-153036; Revised: 23-Nov-2024, Manuscript No. R-153036; Published: 30-Nov-2024, DOI: 10.15421/2024_582
Copyright: This is an open access article distributed under the terms of the Creative Commons Attribution License, which permits unrestricted use, distribution, and reproduction in any medium, provided the original work is properly cited.