Research - (2021) Volume 0, Issue 0
Effectiveness of inductively coupled plasma optical emission spectrometry (ICP OES) in macro and microelements assessment in water
O.M. Chechet1, S.V. Shulyak1, L.V. Shevchenko2, V.M. Mykhalska2*, O.S. Gaidei1, K.S. Miahka1, O.V. Krushelnytska3, N.V. Liniichuk1, I.U. Bardyk1, M.J. Kryvenok2 and S.V.Boyarchuk2Abstract
The suitability of the method was evaluated for determining 15 elements (aluminum, stibium, arsenic, boron, cadmium, chromium, cobalt, copper, iron, lead, manganese, molybdenum, selenium, argentum, sodium, zinc) using atomic emission inductive coupling plasma was evaluated (ICP OES) and defined validation characteristics of the method, namely: detection limit, quantification limit, accuracy, correctness, convergence, reproducibility, selectivity, linearity, and operating range. Modification of the method allowed to exclude the stage of filtration of the sample to reduce the sample preparation time, model the nitric acid content in it, and introduce the use of the internal indium standard, taking into account the proximity of atomic masses of individual elements. This allowed us to level the instrumental drift, select the most favorable emission wavelengths for 10 of the 15 elements, and get a clearer signal and a more accurate result. This method is simple, sensitive, practical, and universal, which is confirmed by LOD and LOQ data. Approbation of this method to determine the content of individual elements in drinking water showed that it demonstrates good selectivity, accuracy, and linearity in the concentration range of 1-1000 μg/l depending on the researched element. Using the method of atomic emission inductively coupled plasma (ICP OES) to determine the content of 15 mineral elements in drinking water, which came to the laboratory from the settlements of Ukraine, showed that in 2020–out of 105 samples in five were found to exceed the MRL of iron in 1.5-2.4 times, in 2021 from the analyzed 58 samples–in one sample the iron content exceeded the MRL almost two times. The got validation data meet the requirements of European directives.
Keywords
Macro, microelements, ICP OES, drinking water, validation.
Introduction
In recent decades, anthropogenic human activity has increased hundreds of times the emissions of heavy metals into the environment, which has led to the ecological decline. Agriculture, industry, engines, waste disposal all contribute to increasing the concentration of toxic elements in the air, soil, food, and drinking water (Dion et al., 2018; Rahman et al., 2017; Voitsitskiy et al., 2019). Heavy metals are toxic to biological objects, and even in ultra-low concentrations, cause chronic diseases in humans and animals (Burke et al., 2016; Khan, 2011; Shahid et al., 2018; Sharma and Bhattacharya, 2017; Natsionalna Dopovid, 2017).
Essential elements in specific quantities are necessary and help maintain biological processes in the organism, but with increasing concentration, they enter metabolic processes, disrupting their functions, and causing some chronic pathologies that accumulate in body tissues (Stanko, 2012; Scheelbeek et al., 2017).
Therefore, the maximum permissible levels of mineral elements in drinking water are regulated (Council Directive 96/23/EC, 1996; DSTU 7525, 2014).
Determining metal ions in tap water and mineral waters with low and high levels of concentration for some elements remains one of the essential tasks of controlling its quality and safety. To solve this task, several methods are used: Atomic Absorption with Flame Atomization (AAS), Atomic Absorption with Electrothermal Atomization (AAS ET), Atomic Emission Spectrometry (ICP EOS), Mass Spectrometry with Inductively Coupled Plasma (ICP MS). Each of these methods has its advantages and disadvantages: different sensitivity, accuracy, and selectivity (Ipeaiyeda and Ayoade, 2017; Płotka-Wasylka et al., 2018; Carvalho, 2015; Massadeh et al., 2020; Shulyak et al., 2021).
The atomic absorption method with flame atomization is not able to provide the appropriate sensitivity and the necessary limits of detection for certain elements (Lead, Cadmium, Arsenic), so its use can lead to misinterpretation of the results of analysis of drinking water samples (Ipeaiyeda and Ayoade, 2017).
The use of atomic absorption spectrometry with electrothermal atomization is appropriate for determining individual elements in water, as it is a one-element analysis. This significantly slows down the measurement process while increasing consumables, which increases the cost of the research. In addition, this method does not have sufficient sensitivity for some elements (B, Se, Sb) (Ipeaiyeda and Ayoade, 2017; Płotka-Wasylka et al., 2018).
ICP MS-is characterized by high sensitivity, wide linear calibration ranges, and simultaneous multi-element quantification. Mass spectrometry provides information about each atom, namely its mass, thereby providing high resolution. However, this method requires expensive equipment and maintenance (Płotka-Wasylka et al., 2018).
The method of optical emission spectrometry with inductively coupled plasma ICP EOS also involves the simultaneous multi-element quantification of metals in a water sample, which significantly speeds up the process of routine analysis (Alexander and Rohman, 2019; Manousi and Zachariadis, 2020).
One of the advantages of this method is the presence of characteristic atomic emission lines generated by the radio-frequency inductively coupled plasma. The spectrum of radiation is decomposed on the diffraction grating of the spectrometer, while detectors record the intensity of the lines. Signals from detectors are monitored and processed by a computer system. An appropriate adjustment method compensates for the variable contribution of background, interference, and interference. The optical spectrum with the possibility of axial and radial view allows to simultaneously determine many elements in the range from 165 to 800 nm (Massadeh et al., 2020; ISO 11885:2007).
The literature describes in sufficient detail the methods of determining macro-microelements in water, but their list sometimes does not correspond to the list of regulated elements by the normative documentation of the Council of Europe 93/86 (Rahman et al., 2017; Burke et al., 2016). Given this, the existing official method of determining DSTU ISO 11885: 2005 required optimization with subsequent validation. The study objective was drinking water, the content of certain mineral elements, the method for determining the elements.
Materials and Methods
The research was carried out in the laboratory of atomic absorption spectrophotometry of the research chemical-toxicological department of the State Research Institute of Laboratory Diagnostics and Veterinary Sanitary Examination. To determine the content of aluminum, stibium, arsenic, boron, cadmium, chromium, cobalt, copper, iron, lead, manganese, molybdenum, selenium, argentum, sodium, zinc in water samples using Optical Emission Spectrometer (ICP-OES) PlasmaQuant PQ(Germany). Ultrapure nitric acid (Merck, Germany), certified multi-element and one-element standard solutions for atomic emission spectrometry (Merck, Germany) with certified ion content were used for preservation of samples and preparation of background, calibration solutions (ISO 11885:2007, 2007; ISO 3696:1987; ISO 5667-3:2003). The solvent was ultrapure deionized water prepared by Atrium 631 UV (Sartorius, Germany). An internal indium standard (Merck, Germany) was used to stabilize the signal and optimize the intensity of the spectral lines of the analytes. Drinking water samples of 100 ml were taken for the research. The purpose of the work is to evaluate the suitability of the method for determining the content of macro and microelements in drinking water using atomic-emission inductively coupled plasma (ICP OES).
Results and Discussion
The validation process for determining the elemental composition of water was provided by examining 80 drinking water samples, including mineral water, and determining 20 samples of certified reference material with certified metal ion content (ERM-CA011c Hard Drinking Water UK-Metals, LGC, UK). Key operating parameters were determined: limit of quantification, the limit of detection, convergence, reproducibility, accuracy, linearity, specificity, stability. The work results were processed according to the accepted criteria (statistical processing of the obtained data was performed in the program MS Excel, 2013).
As a result of the work, the preparation of samples for analysis was also optimized-the filtration stage was excluded, which does not give the expected result because the existing inclusions of drinking water do not affect the supply of the sample through the sprayer to the cyclic chamber and the quality of the analysis.
To eliminate possible non-spectral interference (suppression of elemental signals due to the presence of acid in the solution and the content of various salts) in this technique was simulated the content of nitric acid in the sample for analysis as follows, that the concentration of the matrix of working calibration standards and the background solution corresponds to the concentration of the test sample, which is 2% HNO3 (2 ml of concentrated HNO3 per 100 ml of water). According to the standardized method, the sample is acidified with 0.5% HNO3 solution). In general, the sample preparation period has decreased.
To eliminate spectral interference in this method, the internal indium standard was introduced, based on the proximity of the atomic masses of some of the studied elements, which allowed to level the instrumental drift.
Working Range or interval-evaluated by checking the ability of the analytical method to ensure accuracy, correctness and linearity in the research of samples containing the analyte at the boundary of the interval and within it. The area where the results have acceptable uncertainty; the data are shown in Table 1. The lower limit of the operating range was determined by the limit of quantification, and the upper-the point where the level of change of reaction per unit change of concentration is insufficient, as shown in Fig. 1.
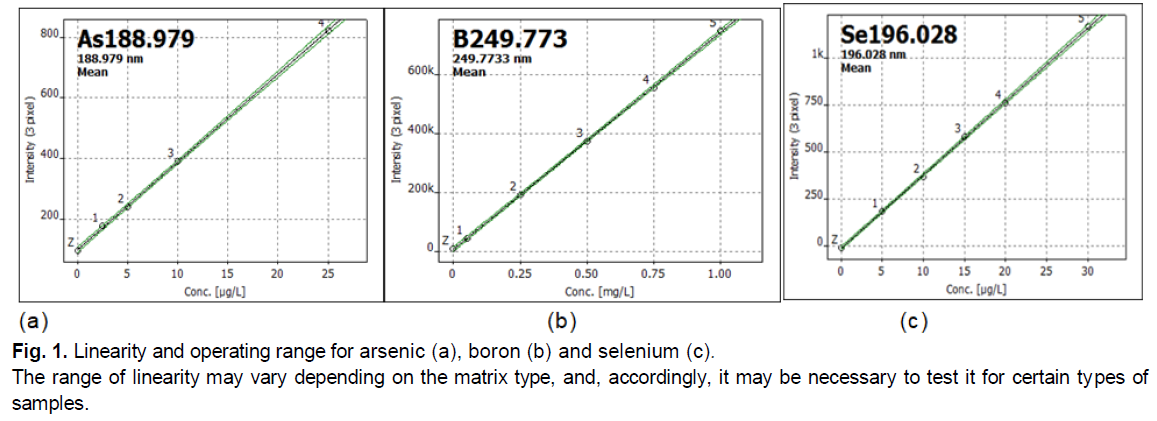
Figure 1: Linearity and operating range for arsenic (a), boron (b) and selenium (c).
Element Name | Working Range, μg/l | Element Name | Working Range, μg/l |
---|---|---|---|
Al | 10-200 | B | 500-1000 |
As | 1-20 | Cd | 2-100 |
Sb | 1-20 | Cu | 100-2000 |
Cr | 5-100 | Pb | 1-20 |
Co | 5-100 | Se | 1-20 |
Mn | 5-100 | Zn | 50-1000 |
Mo | 5-100 | Na | 5000-25000 |
Fe | 10-200 |
Table 1. Working range of the research elements.
The range of linearity may vary depending on the matrix type, and, accordingly, it may be necessary to test it for certain types of samples.
Fig. 2 shows, for example, the signals on these emission lines for arsenic, selenium, and boron.
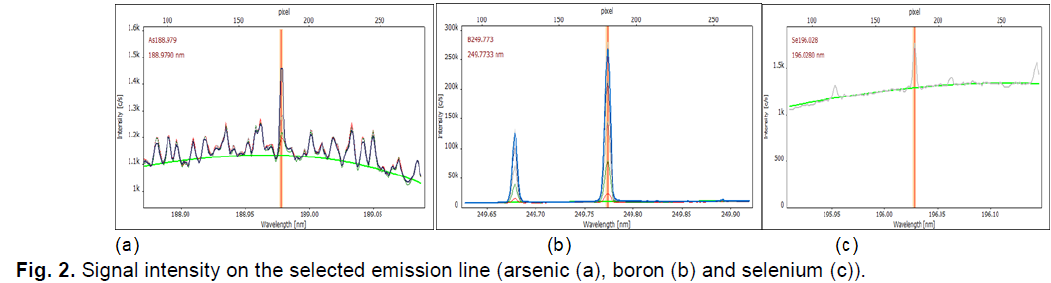
Figure 2: Shows, for example, the signals on these emission lines for arsenic, selenium, and boron.
Linearity and working range allow a more accurate assess the results. Linearity-is the ability to show that the test results are proportional to the analyte concentrations within a given range set for the method. The slope of the regression line and its variation give a mathematical degree of linearity. Linearity was assessed by visually checking the graph of the measuring calibration samples. The data of standard curves for the working range of the listed elements are linear, as shown in the example of Fig. 1.
Accuracy, Trueness was determined using certified reference material, as it characterizes the closeness of the results to the actual value and evaluated by the error of determination. Furthermore, it was accepted as a quantitative difference between the average of a set of results or an individual result and the value taken as the actual (assigned) value for the measured quantity (Table 2).
Element | The Wavelength of Emission Provides a Standardized Method nm | The Proposed Emission Wavelength, nm | The results of the Accuracy of the Method When Using New Emission Waves | |||
---|---|---|---|---|---|---|
Certified Value ERM-CA011c, μg/l | The actual Value of μg/l | Accuracy, % | Uncertainty, μg/l | |||
Mn | 257.610 | 259.372 | 49.10 | 48.50 | 98.70 | 1.60 |
As | 193.690 | 188.979 | 10.25 | 10.02 | 97.95 | 0.41 |
Al | 308.215 | 309.271 | 199.70 | 185.90 | 93.88 | 7.50 |
Sb | 182.036 | 181.975 | 15.25 | 14.62 | 95.86 | 2.30 |
Cr | 205.552 | 206.158 | 49.30 | 48.91 | 99.21 | 1.90 |
Co | 228.616 | 238.890 | 5.25 | 5.15 | 98.09 | 0.21 |
Mo | 202.030 | 202.030 | 5.32 | 5.11 | 96.05 | 0.31 |
B | 208.950 | 249.773 | 993.00 | 991.02 | 99.80 | 48.00 |
Cd | 214.438 | 214.438 | 5.31 | 5.15 | 96.98 | 0.23 |
Cu | 324.754 | 224.702 | 1993.00 | 1989.10 | 99.80 | 62.00 |
Pb | 220.353 | 220.353 | 10.01 | 9.56 | 99.80 | 0.17 |
Se | 196.026 | 196.028 | 11.13 | 10.91 | 98.90 | 0.47 |
Zn | 206.191 | 206.191 | 605.00 | 603.55 | 99.76 | 17.00 |
Fe | 259.940 | 239.562 | 198.30 | 196.35 | 96.97 | 5.10 |
Na | 589.592 | 589.592 | 24.47 | 23.87 | 97.54 | 0.53 |
Table 2. Accuracy and operating parameters of the optical system (emission wavelength) for each element.
The minimum accuracy of the quantitative method according to Council Directive 96/23 EU on analytical methods and the interpretation of results (Commission Decision 2002/657/EC, 2002) is shown in Table 3. As can be seen from the above data, the method's accuracy fully complies with regulatory requirements.
Analyte Concentration | Regulated Value |
---|---|
≤ 1 μg/kg | - 50% to+20% |
>1 μg/kg to 10 μg/kg | - 30% to+10% |
≥10 μg/kg | - 20% to+10% |
Table 3. Regulated values of accuracy.
Repeatability was determined by multiple (10-20) measurements of a suitable homogeneous sample of mineral water with a known analyte content under the same conditions, resulting in evaluating the closeness of the results to each other. This is an indicator of short-term fluctuations of research results, the criterion of which is the standard deviation of parallel values.
If the content of analytes in the water sample is in the range of 0.001–0.5 mg/kg, the acceptable value of RSD is not >20%. In other cases, the value of RSD not >5 is considered acceptable.
Reproducibility was determined by repeated examination of samples under different conditions, as it is a characteristic of the quality of measurement results, which reflects the closeness of the results of repeated observations in different conditions (Table 4).
The Name of the Component | Mass Fraction, μg/l | Intra-laboratory Reproducibility, R, % (normative) | Intralaboratory Reproducibility, R, % (actual) | Convergene, R, % |
---|---|---|---|---|
Mn | >0.01-0.1 | 20 | 11.1 | 5.2 |
Mn | >0.10-1.0 | 15 | 8.20 | 2.3 |
Mn | >1.0 | 10 | 5.80 | 2.1 |
Al | >0.01-0.1 | 20 | 10.0 | 6.2 |
Al | >0.10-1.0 | 15 | 7.55 | 2.2 |
Al | >1.0 | 10 | 5.02 | 2.1 |
Sb | >0.01-0.1 | 20 | 12.0 | 6.3 |
Sb | >0.10-1.0 | 15 | 7.51 | 4.2 |
Sb | >1.0 | 10 | 4.2 | 1.9 |
Cr | >0.01-0.1 | 20 | 10.1 | 4.5 |
Cr | >0.10-1.0 | 15 | 7.2 | 2.3 |
Cr | >1.0 | 10 | 5.1 | 2.1 |
Co | >0.01-0.1 | 20 | 12.2 | 5.1 |
Co | >0.10-1.0 | 15 | 7.5 | 2.5 |
Co | >1.0 | 10 | 4.6 | 1.9 |
Mo | >0.01-0.1 | 20 | 14.3 | 5.5 |
Mo | >0.10-1.0 | 15 | 12.0 | 4.2 |
Mo | >1.0 | 10 | 5.9 | 4.1 |
B | >0.01-0.1 | 20 | 9.5 | 4.9 |
B | >0.10-1.0 | 15 | 7.1 | 2.5 |
B | >1.0 | 10 | 4.2 | 2.3 |
Cd | >0.01-0.1 | 20 | 11.3 | 4.5 |
Cd | >0.10-1.0 | 15 | 10.2 | 2.8 |
Cd | >1.0 | 10 | 6.4 | 2.1 |
Cu | >0.01-0.1 | 20 | 12.1 | 2.5 |
Cu | >0.10-1.0 | 15 | 7.8 | 2.1 |
Cu | >1.0 | 10 | 4.5 | 1.7 |
Pb | >0.01-0.1 | 20 | 12.0 | 4.6 |
Pb | >0.10-1.0 | 15 | 5.9 | 3.1 |
Pb | >1.0 | 10 | 9.5 | 3.1 |
Se | >0.01-0.1 | 20 | 11.4 | 5.1 |
Se | >0.10-1.0 | 15 | 7.8 | 3.8 |
Se | >1.0 | 10 | 4.5 | 2.4 |
Zn | >0.01-0.1 | 20 | 12.2 | 3.6 |
Zn | >0.10-1.0 | 15 | 10.1 | 2.1 |
Zn | >1.0 | 10 | 5.5 | 2.3 |
Na | >0.01-0.1 | 20 | 12.5 | 6.1 |
Na | >0.10-1.0 | 15 | 8.4 | 4.5 |
Na | >1.0 | 10 | 4.6 | 3.6 |
Fe | >0.01-0.1 | 20 | 9.5 | 4.1 |
Fe | >0.10-1.0 | 15 | 3.4 | 2.1 |
Fe | >1.0 | 10 | 2.6 | 2.2 |
As | >0.01-0.1 | 20 | 11.1 | 5.3 |
As | >0.10-1.0 | 15 | 8.4 | 4.4 |
As | >1.0 | 10 | 7.5 | 3.8 |
Table 4. Intra-laboratory reproducibility and convergence of the researched elements.
Insufficient stability of the test substance or matrix elements in the sample during storage or analysis can cause significant deviations in the analysis results, so the stability of the calibration standard in solution and the matrix-water was determined. The stability of the test substance was well characterized under different storage conditions. Therefore, for its determination, the primary standard analyte solution was prepared and dissolved to get different concentrations (from the minimum corresponding to the detection limit). Forty aliquots were taken from each concentration and examined for analyte content immediately after preparation. Distributed equal amounts of samples in appropriate containers, labeled them, and stored them at temperatures: 4°C, 20°C, and -20°C for a month. The reproducibility of the research results complied with European Council Directive 2002/657.
Stability in the matrix was determined using certified reference material.
This material was divided into four aliquots; one of the aliquots was examined immediately after cooking in fresh material; in others, the concentration of the analyte was determined after 1, 2, and 4 weeks. As the research results showed, the concentration of analytes in the samples did not change during the specified storage period, which confirms their stability.
The specificity or selectivity of the method was accepted by the extent to which it provides unambiguous detection and determination of a particular analyte in the mixture without mutual influence from other components that can be expected in the matrix (impurities, closely related chemical compounds, decomposition products, placebo ingredients). No severe interactions have been reported.
The limit of detection or discovery of the analyte's analytical method (Limit of Detection, LOD) was determined by re-analysis 20 blank (zero) sample samples. The detection limit is statistically defined as some factor equal to the doubled (two-sigma criterion) or tripled (three-sigma criterion) standard deviation. This number is numerically equal to twice the standard deviation of 2S0.
To assess the limit of quantification of LOQ (Limit of Quantitation) (the lowest level of analyte content in the matrix sample) was performed a 10-fold analysis of test samples with low analyte concentration (Table 5).
Element, μg/l | LOD, Detection Limit | LOQ, Quantification Limit | Maximum Allowable Levels, According to DSTU 7525: 2014 | Maximum Permissible Levels According to Council Directive 98/83/EC |
---|---|---|---|---|
Mn | 0.4 | 2.0 | 50 | 50 |
As | 0.4 | 2.0 | 10 | 10 |
Al | 1.0 | 5.0 | 200 | 200 |
Sb | 0.2 | 1.0 | 5 | 5 |
Cr | 0.4 | 2.0 | 50 | 50 |
Co | 0.4 | 2.0 | - | - |
Mo | 0.4 | 2.0 | - | - |
B | 20.0 | 100.0 | 500 | 1000 |
Cd | 0.4 | 2.0 | 1 | 5 |
Cu | 0.6 | 3.0 | 1000 | 2000 |
Pb | 0.2 | 1.0 | 10 | 10 |
Se | 0.2 | 1.0 | 10 | 10 |
Zn | 2.0 | 10.0 | - | - |
Fe | 0.4 | 2.0 | 200 | 200 |
Na | 10.0 | 100.0 | 200 | 200 |
Table 5. The results of determining the Detection limit (LOD) and the limit of Quantification (LOQ).
LOQ was calculated as the analyte concentration equal to the standard deviation (s0′) at low concentration levels multiplied by the coefficient k. The IUPAC Recommendation assumes a default value of k=10, and if the standard deviation is approximately constant at low analyte concentrations, this factor corresponds to a Relative Standard Deviation (RSD) of 10%. Multipliers 5 or 6 are also sometimes used, then RSD values are 20% and 17%, respectively. According to Council Regulation 2007/333/EC, the limit of quantification for atomic absorption spectrometric methods should not exceed 1/5, and for lead, 2/5 of the maximum level specified in Council Regulation 2006/1881/EC. Maximum levels of heavy metals, macro- and microelements in drinking water in Ukraine are regulated by DSTU 7525: 2014 and European Council Directive 98/83. Therefore, an important criterion is the value of the limit of quantification of the method for correct interpretation and reliability of the analysis results.
Using the method of atomic emission inductively coupled plasma (ICP OES) to determine the content of 15 mineral elements in drinking water, which came to the laboratory from the settlements of Ukraine, showed that in 2020-out of 105 samples in five were found to exceed the MRL of iron in 1.5-2.4 times, in 2021 from the analyzed 58 samples-in one sample the iron content exceeded the MRL almost two times.
Conclusion
The results got during validation demonstrate that this method is fast, sensitive, accurate when used in routine researches of drinking water, according to the normative values of Council Directive 98/83 EU, and has sufficient levels of LOD and LOQ that meet the requirements of the European Directive 2002/657EC. The ICP OES method for determining the content of individual macro-, microelements, and heavy metals in water is easy to use, with no concentration and sample preparation and allows to determine the content of individual elements in a wide range of concentrations in water samples, which is essential for the interpretation of data on the quality and safety of drinking water of centralized water supply, mineral and artificially enriched with trace elements water. This allows to use of the ICP OES method for its intended purpose and gets high-precision data.
References
Alexander, D., Rohman, A. (2019). Analitykal metod validation of icp-aes for analisis of cadmium, chromium, cuprum, mangan, and nikel in milk. Journal of Applied Pharmacy, 11:341-344.
Burke, F., Hamza, S., Naseem S., Nawaz-ul-Huda S., Azam M., Khan I. (2016). Impact of cadmium polluted groundwater on human health: winder, balochistan. SAGE Open.
Carvalho, R.N.C.S., Brito, G.B., Korn, M.G.A., Teixeira J.S.R., Dias F.de.S., Dantas A.F., Teixeira L.S.G. (2015). Multi-element determination of copper, iron, nickel, manganese, lead and zinc in environmental water samples by ICP OES after solid phase extraction with a C18 cartridge loaded with 1-(2-pyridylazo)-2-naphthol. Analytical Methods, 20:1-6.
Commission Decision. (2002). Implementing Council Directive 96/23/EC concerning the performance of analytical methods and the interpretation of results. Journal of European Communication.
Commission Regulation (EC). (2007). Laying down the methods of sampling and analysis for the official control of the levels of lead, cadmium, mercury, inorganic tin, 3-MCPD and benzo(a)pyrene in foodstuffs (Text with EEA relevance) Special edition in Croatian.
Directive, C. (1996). Council Directive 96/23/EC of 29 April 1996 on measures to monitor certain substances and residues thereof in live animals and animal products and repealing Directives 85/358/EEC and 86/469/EEC and Decisions 89/187/EEC and 91/664/EEC. OJ EC L, 125:10-31.
Directive, C. (1998). 98/83/EC of 3 November 1998 on the quality of water intended for human consumption. Official Journal of the European Communities.
Dion, L.A., Saint-Amour, D., Sauvé, S., Barbeau, В., Mergler, D., Bouchard, M. ( 2018). Changes in water manganese levels and longitudinal assessment of intellectual function in children exposed through drinking water. Neurotoxicology, 64:118-125.
Voda, P. (2014). Vymohy ta metody kontroliuvannia yakosti. Kyiv: Derzhspozhyvstandart (in Ukrainian).
Ipeaiyeda, A.R., Ayoade, A.R. (2017). Flame atomic absorption spectrometric determination of heavy metals in aqueous solution and surface water preceded by co-precipitation procedure with copper (ii) 8-hydroxyquinoline. Applied Water Science, 7:4449–4459.
ISO. (2007). Water quality–determination of selected elements by inductively coupled plasma optical emission spectrometry (ICP-OES). Second edition 2007-08.
ISO. (1987). Water for analytical laboratory use–Specification and test methods First edition.
ISO. Water quality–Sampling-Part 3:Guidance on the preservation and handling of water samples. Third edition 2003-12.
Khan, T. (2011). Trace elements in the drinking water and their possible health effects in aligarh city, India. Journal of Water Resource and Protection, 3:522-530.
Manousi, N., Zachariadis, G.A. (2020). Development and application of an icp-aes method for the determination of nutrient and toxic elements in savory snack products after autoclave dissolution. Separations, 7:66.
Massadeh, A.M., El-Rjoob, A.W.O., Gharaibeh, S.A. (2020). Analysis of selected heavy metals in tap water by inductively coupled plasma-optical emission spectrometry after pre-concentration using chelex-100 ion exchange resin. Water, Air, and Soil Pollution, 231:1-14.
http://www.minregion.gov.ua/wp-content/uploads/2017/12/Proekt-Nats.-dop.-za-2016-rik.pdf
Płotka-Wasylka, J., Frankowski, M., Simeonov, V., Polkowska Ż., Namieśnik J. (2018). Determination of Metals Content in Wine Samples by Inductively Coupled Plasma-Mass Spectrometry. Molecules, 23:2886.
Rahman, S.M., Kippler, M., Tofail, F., Bölte S., Hamadani J.D., Vahter M. (2017). Manganese in drinking water and cognitive abilities and behavior at 10 years of age: a prospective cohort study. Environmental Health Perspectives, 125:057003.
Scheelbeek, P.F.D., Chowdhury, M.A.H., Haines, A., Alam D.S, Hoque A.M., Butler А.Р., Khan E.A., Mojumder K.S., Blangiardo A.G.М., Elliott Р., Vineis Р. (2017). Drinking water salinity and raised blood pressure: evidence from a cohort study in coastal Bangladesh. Environmental Health Perspectives, 125:057007.
Shahid, M., Niazi, N.K., Dumat, C., Naidu R., Khalid S., Rahman M.M., Bibi I. (2018). A Meta-analysis of the distribution, sources and health risks of arsenic-contaminated groundwater in Pakistan. Environmental Pollution, 242:307-319.
Sharma, S., Bhattacharya, A. (2017). Drinking water contamination and treatment techniques. Applied Water Science, 7:1043.
Shulyak, S., Shevchenko, L., Mykhalska, V., Kaminska, O., Gaidei, O., Kovalenko, V, Balatskyi, Y., Кryvenok, M., Boyarchuk, S. (2021). Quality and safety of tap water in selected regions of Ukraine. Ukrainian Journal of Ecology, 11:274-283.
Stanko, O.M. (2012). Vazhki metaly u vodi: zabrudnennia richky dnister za ostanni 10 rokiv (terytoriia Lvivskoi oblasti). Suchasni Problemy Toksykolohii, 3:58-63 (in Ukrainian).
Voitsitskiy, V.M., Danchuk, V.V., Ushkalov, V.O., Midyk, S.V., Kepple, O.Yu., Danchuk, О.V., Shevchenko, L.V. (2019). Migration of antibiotics residual quantities in aquatic ecosystems. Ukrainian Journal of Ecology, 9:280-286.
Author Info
O.M. Chechet1, S.V. Shulyak1, L.V. Shevchenko2, V.M. Mykhalska2*, O.S. Gaidei1, K.S. Miahka1, O.V. Krushelnytska3, N.V. Liniichuk1, I.U. Bardyk1, M.J. Kryvenok2 and S.V.Boyarchuk22National University of Life and Environmental Sciences of Ukraine, 15 Heroiv Oborony St., Kyiv, 03041, Ukraine
3Stepan Gzhytskyi National University of Veterinary Medicine and Biotechnologies, 50 Pekarska St., Lviv, 79010, Ukraine
Citation: Chechet, O.M., Shulyak, S.V., Shevchenko, L.V., Mykhalska, V.M., Gaidei, O.S., Miahka, K.S., Krushelnytska, O.V., Liniichuk, N.V., Bardyk, I.U., Kryvenok, M.J., Boyarchuk, S.V. (2021). Effectiveness of inductively coupled plasma optical emission spectrometry (ICP OES) in macro and microelements assessment in water. Ukrainian Journal of Ecology 11 (7), 197-203.
Received: 18-Aug-2021 Accepted: 25-Sep-2021 Published: 30-Sep-2021
Copyright: This is an open access article distributed under the terms of the Creative Commons Attribution License, which permits unrestricted use, distribution, and reproduction in any medium, provided the original work is properly cited.