Research - (2023) Volume 13, Issue 5
Bioremoval and resource recovery of nutrients by phytoremediation using aquatic free floating plants Lemna minor
K. Usharani1,2* and V. Arunkumar1Abstract
The contamination of water resources by phosphate, sulphate, nitrate along with iron from various sources, those wastewater cause a severe environmental problem. The aquatic free floating plants, L. minor has been revealed to be a noble model for eco-toxicological studies and it has the capability to bioaccumulate or biosorption of nutrients and metals. The security of drinking water is a very vital regarding the public health issue. However the iron (Fe) is only toxic at very high concentrations and it acts as a valuable substitute for other heavy metals and its existence in drinking water is an actually unsafe to public health. The work intended to test the potential of the aquatic free floating plant species L. minor to phytoremediate the iron rich wastewater, through bioreduction of Iron under laboratory conditions and to evaluate the time required for the maximum removal of Iron, also biosorption or bioremoval of phosphate, sulphate and nitrate. The purpose of the study was to evaluate the efficiency of bioremoval of nutrients (phosphate, sulphate and nitrate) and bioreduction of iron (Fe+3 ions) from aqueous solution by Lemnna minor under different conditions of adsorbent dosage, initial concentration, pH and contact time along with the nature of biosorption of iron. The results have shown that Lemnna minor was able to grow and develop in the Fe-rich wastewater and having potential in the bioremoval of the Fe element. Throughout the 21 days of testing it was found that there was a significant increase in the biomass of Lemnna minor both in the contaminated and in the non-contaminated waters. It was furthermore found to be that bioremoval of nutrients (phosphate, nitrate and sulphate) by biosorption or bioaccumulation of Fe occurred mainly during the first 7 days of experimental analysis. It was found that L. minor has potential and bioprospecting for the biotreatment and biorecovery of effluents rich in iron.
Keywords
Eutrophic lake water, Nutrient recovery, Free floating aquatic duckweed, Phytoremediation.
Introduction
The iron is unique and vital elements in trace concentration for human life and its environment. The daily requirement of iron is in range 1-2 mg/day of which 75% are found in the blood and the rest 25% are in the bone marrow and liver (Prashanth, et al., 2015). Iron has biochemically available valence status that plays a vital role in a wide variety of electron transference processes and enzymatic actions (Bourzama, et al., 2021). The occurrence of iron in water plays an significant part as it improves the growth of iron reducing bacteria that aid in the conversion of iron (II) to iron (III) via oxidation (WHO, 2017). The safety of drinking water is a precise significant to public health issue. However, the iron is only toxic at very high dosage or concentrations, it acts as a useful substitute for other heavy metals and its existence in drinking water is a real unsafe to public health. The rainwater when it infiltrates the soil and underlying geologic formations runs iron, causing it to seep into aquifers that aid as sources and causes of iron in groundwater for wells. Although it present in drinking water, iron is seldom found at reddish brown color and iron content is generally present in natural waters either in ferrous or ferric state depending on its pH value and dissolved oxygen. The presence of iron in natural waters can be attributed to the weathering of rocks and minerals, acid mine drainage, sewages or iron related industries. The iron may also be contributed to groundwater by its contact with well casing, pump assemblies, pipes and storage tanks. However, it has high values because at overdose it causes several health problems includes as anorexia, diarrhea, diphasic shock, metabolic acidosis, vascular congestion of the gastrointestinal tract, brain, spleen and thymus, and death (Marsidi, et al., 2018).
In the ecosystem, the iron exists in two forms, which includes the soluble ferrous iron and insoluble ferric particulate iron (Marsidi, et al., 2018). It is usually found in natural fresh water but its concentration differ conferring to geographical location (Joe-Wong, et al., 2017). The geotechnique profile of ecosystems is the core factor controlling groundwater hydrology. Also the iron is establish in rocks and soil under appropriate conditions which will leach into the water resources from rock and soil formations. The contamination of iron in groundwater arises naturally or by anthropogenic causes together with industrial effluents, landfill leakage and acid mine drainage. Moreover well casings, pump components, pipes and storage tanks can also contribute to Fe ion in water leads to contamination (Marsidi, et al., 2018; Ajiboye, et al., 2019; Su, et al., 2019; El-Beltagi, et al., 2020; Wang, et al., 2020). The water with more dosage Fe+3 can stain clothes, dishware, discolor plumbing fixtures, furthermore from time to time add a rusty look and tasty to the water. The exceeding level of iron (Fe+3) concentrations yield a yellow to reddish form in water. When the concentration of iron level in water is right high, it developed further toxic and reason for several dilemmas for human health. Therefore, iron need be uptake or altered to less toxic form in water before applied for irrigation or being discharged to the ecosystem. In all over the world, there are no health-based guidelines for the iron concentration in drinking water standard. Though, based on taste and nuisance concerns the permissible limit for iron in drinking water is (0.3 mg/l) according to the World Health Organization (WHO) and Environment Protection Agency (EPA, 2019). The enlarged in the urbanization and industrialization activities such as electroplating, steel manufacturing, wood preservation, tanning and glass manufacturing lead to upturn the pollutants, metals, heavy metals, other hazardous compounds into the water and contaminated the corresponding environment (Brusseau, et al., 2019; Cao, et al., 2018; Olawale, et al., 2021; Qasem, et al., 2021; Zgłobicki, et al., 2022; Zhang and Duan, 2020). The water pollution followed by changes in the significant physico-chemical and biological parameters of water that has a hazardous impact on environment and its human health (Rajeshkumar, et al., 2018; Schneider, et al., 2019).
The presence of these pollutants in the environment or water exposed to the human to many severe diseases including autoimmune disorder, digestive disorder, heart disorder, liver, kidney, stomach and lung cancer. The removal of heavy metal include iron from water is an essential matter. The iron in water often originates from the corrosion of underground iron pipelines, including wells and bore wells. Moreover, the iron from the contiguous soil environment can seep in when the soil is saturated. The environmental protection agency (EPA) limitation for iron as 0.3 mg/L, however even lower levels can too cause some of the problems. The technical approaches includes, filtration and an ion exchange are commonly used to clean the water from iron and other harmful substances. The iron (III) bio-reduction deals an assessment of applying the iron redox cycle to nitrate removal or remediata in the aquatic environment (Yongsheng Lu, et al., 2021).
The phytoremediation is the process of using plants and associated soil microorganisms to reduce the concentrations or its toxic effects of contaminants present in the ecosystem. The phytoremediation is widely accepted as a cost-effective environmental restoration technology and are different procedures are available for various environments and its types of contaminants. These involved the different processes such as in situ stabilization or biosorption, degradation and bioremoval. The aquatic plants used in this process of phytotechnology is a cost-effective and also resourceful clean up technique for phytoremediation (act as a natural absorber for environmental contaminants and heavy metals) of a large contaminated area (Pratas, et al., 2014). The elimination of numerous heavy metals along with other contaminants by aquatic plants, which is the most proficient and profitable method (Ali, et al, 2013 and Guittonny-Philippe, et al., 2015). The constructed wetlands using with aquatic plants were broadly applied throughout the world for the treatment of wastewater (Mesa, et al, 2015; Gorito, et al., 2017). The choice and selection of aquatic plant species for the accumulation of heavy metal is a precise substantial matter to enhance the process of phytoremediation (Fritioff and Greger, 2003; Galal, et al., 2018). The aquatic plants always advance an extensive system of roots that helps them and makes them the paramount route for the accumulation of contaminants in their roots and shoots (Mays and Edwards, 2001; Stoltz, et al, 2002). The growth and the cultivation of aquatic plants are time-consuming, which could restrict the growing demand of phytoremediation (Said, et al., 2015). Nevertheless, this shortcoming is substituted by the number of advantages that this technology possesses for the treatment of wastewater (Syukor, et al., 2014; Koźmińska, et al., 2018).
Among the various aquatic plants, the duckweed species are more suitable for iron bioremoval, which are small floating aquatic plants that can found in worldwide develop a thick blanket-like mats on still or slow moving nutrient-rich fresh water and brackish waters. The duckweeds are monocotyledons and their botanical family was Lemnaceae belongs to higher plants or macrophytes they are often mistaken for algae. The duckweeds are able to grow in water at temperatures ranges from 6°C to 33°C and even many species of duckweed stay alive with low temperatures, moreover by forming a turion besides the plant sinks to the bottom of a lagoon and where it remains dormant until warmer this leads to the water brings about a continuation of normal growth. The precise turion yield (STY; number of turions formed by one frond) and the time of onset of turion formation have been used as more specific measures of turion induction (Appenroth, 2002). Though associating with other aquatic free floating duckweed plant leaves have little fiber (5% in dry matter of cultivated plants), also they do not need to support upright erections and structures. The benefit of plant has little and or no indigestible material even for monogastric animals, and this differences with other crops includes soya beans, rice and maize having approximately 50% of the biomass is in the form of high fiber with low digestibility remains. The duckweed plants adapted to an extensive diversity of geographic and climatic zones. They are also establish in all but waterless deserts and enduringly frozen areas. They grow highest in tropical and temperate zones, many species can survive at extreme temperatures. The natural habitat of duckweed species is the surface fresh water and brackish water, these waters act as sheltered from wind and wave action, moreover they do not survive in fast moving water (>0.3 m/second). The water unsheltered from wind is a significant attribute as they do not become weeds in water ways. The assessment of the potential of the duckweed Lemnna minor in treating iron contaminated wastewater through the process of phytotreatment or phytoremediation.
The objectives of the present study was to investigate the potentiality of Lemnna minor (duckweed) for the removal of iron from wastewater, estimation of biomass and total chlorophyll and was focussed for the biosorption property (one of the physicochemical properties of Lemnna minor). Moreover, the purpose was to study the efficiency of bioremoval or biosorption and bioreduction of iron (Fe+3 ions) from aqueous solution by Lemnna minor under different conditions of adsorbent dosage that is the initial iron concentration, pH and contact time along with the nature of biosorption of iron by Lemnna minor species were studied.
Materials and Methods
Sampling location
The Kurichi Lake has a water extent area of 343.96 acres and clenches the least water storage volume since of its shallowness. In this water tank, 9.50 acres is influenced by around 200 huts on the bund and 274 abutting the channels.
The wetland, Kurichi Lake (Fig. 1) received municipal sewage, also it act as a site for dumping garbage from inhabitations around its vicinity. The district of Coimbatore (Manchester of South India), which is the second largest city in the Indian state of Tamil Nadu. It is located at 11°1' 6'' N, 76° 58' 21'' E.
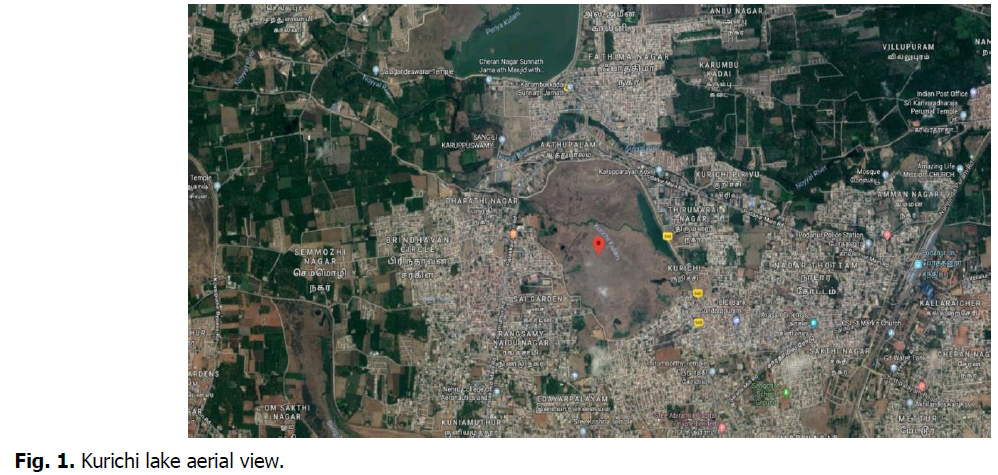
Fig. 1. Kurichi lake aerial view.
The industrialization and urbanization exhibit chief impact on surface water environment in Coimbatore district, Tamil Nadu, India. Owing to the developmental activities both surface and subsurface water sources are receiving pollutants and polluted. There were numerous textile, automobiles, home appliances and small scale industries are located in and around Coimbatore district. The effluents from these industries prominently disturb the water quality of surface water bodies. Therefore, the eutrophic lake in this district of Coimbatore was particular reason for this study area and to analysis various significant physicochemical characteristics of lake water.
Significance of the study on Kurichi lake water
The Small Industries Development Corporations (SIDCO) are the state-owned companies and agencies in the states of India that were established at different times under the policy of Government of India for the promotion of small scale industries. This SIDCO-industrial cluster, is roughly estimated that the total quantum of sewage generated from all industries will be 250 to 300 KL of Sewage per day (cpcb.nic.in). All the units have provided septic tank and arrangements for the disposal of sewage generated from the industrial premises. One unit (M/s. Indo shell Cast Pvt. Ltd.) has provided STP. The sewage disposal from the various individual households and the colonies of various houses are the main sources of pollutants to the surface water located near to the CEPI area (Comprehensive Environmental Pollution Index, monitoring for PIAs (Polluted Industrial Areas). The nearest Surface Water Sampling location is Kurichi Lake which is located at 2.5 km away from the SIDCO industrial estate. Here, there are about 17 foundry located which is major producer of iron casting metals so there is a possible of iron waste in nearby lake Kurichi So, Kurichi Lake was chosen as our study area.
Phytotreatment of phosphate, nitrate, sulphate and fluoride bioremoval from wastewater from Eutrophic Lake using L. minor
The duck weed samples were collected from surface water (Eutrophic Lake waters,Kurichi lake, TN, IN) and the samples were collected in sterilized glass bottle. The collected samples were analyzed for significant physicochemical parameters such as pH, Biochemical Oxygen Demand, Chemical Oxygen Demand, Total Hardness, Phosphate, Nitrate, Sulphate, Iron and Fluoride. The instruments were used precisely and accurately. Analytical grade AR grade chemicals were used in the study. These water quality parameters were determined following the Standard Methods (APHA, 2007).
The study was conducted in the reactors (500 mL), planted with L. minor plants, with 5 g of L. minor added to each of the reactors. Then 500 mL of solutions with different concentrations from 10 to 120 ppm of various Nutrients (iron, phosphate, sulphate, nitrate and fluoride) were placed inside the reactor containers, beside the experiment was conducted with the eutrophic wastewater and control with deionized water were used in the study. In the reactors the L. minor were harvested in various hydraulic retention times (HRTs). Afterwards the concentrations of iron, phosphate, sulphate, nitrate and fluoride were determined using UV-Visible spectrophotometer (UV-2600 series SHIMADZU).
Then the wastewater phytotreated samples were allowed to cool and measured the absorbance in terms of optical density (OD) at 510 nm for Iron, 690 nm for Phosphate, 540 nm for Nitrate, 410 nm for Sulphate, 544 nm for Fluoride using a UV-Visible spectrophotometer (UV-2600 series SHIMADZU). The initial and residual concentration of iron, phosphate, nitrate, sulphate and fluoride were measured in terms of optical density (OD) value using UV-Visible spectrophotometer. From this value, the percentage reduction or removal of each elements and nutrients were calculated. The elements and nutrient uptake efficiency (E) were calculated using the formula, E=[(I-F)/I] × 100/ growth of plants (biomass). Where, I and F are the Initial and Final concentrations of nitrate respectively. The growth of the plant in terms of biomass and chlorophyll contents were measured.
An efficiency value of 100% was obtained when no target chemical species appeared in the treated water sample (i.e., F=0) (Usharani, et al., 2011, 2013, 2017; Usharani and Keerthi, 2020). The change in pH observed in treated wastewater at different concentrations after treatment by the L. minor (free floating aquatic plants) were estimated using digital pH meter (Scientific Tech, Advanced pH meter, mode ST-2002).
Phytotreatment of Iron bioremoval from wastewater from Eutrophic Lake using L. minor
The wastewater (Eutrophic wastewater) and control (deionized water) were used in the iron phytoremediation process using Lemnar minor (duckweed). Iron with different concentrations (up to 100 ppm) were prepared and 6 gm of wet biomass of duckweed was used as an initial seed culture in the phytotreatment study (Fig. 2). To the serious of standards, blank and 10 ml of sample, 5 ml of each 1, 10-phenanthroline monohydrate and acetate buffer was added and made up to 50 ml with distilled water. After five minutes, the intensity of the color was measured at 510 nm spectrophotometrically. Iron content in the water sample was calculated from standard graph respectively.
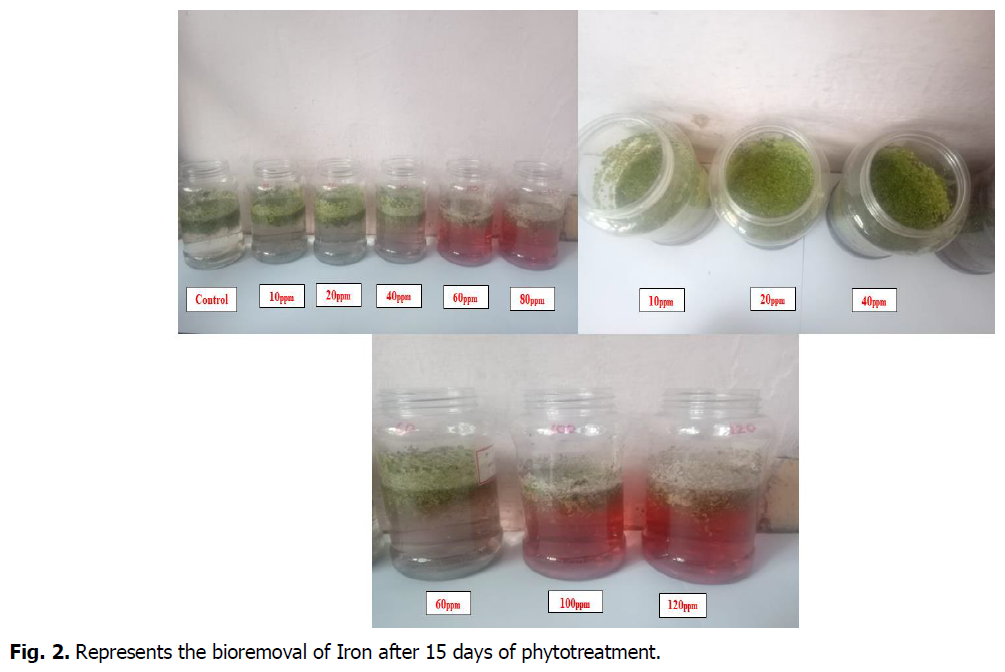
Fig. 2. Represents the bioremoval of Iron after 15 days of phytotreatment.
The Phosphorus species is a most common component of agricultural fertilizers, manure, organic wastes in sewage and industrial effluents. Also, it is a vital element for plant life, however when there is too abundant of it in water can speed up the eutrophication, which leads to a reduction in dissolved oxygen in water bodies caused by an increase of mineral and organic nutrients of rivers, lakes and ponds. The soil erosion is a main contributor of phosphorus to streams. This Phosphorus element can be measured calorimetrically at 690 nm using spectrophotometer. To the series of standards, blank and sample, 4 ml of ammonium Molybdate solution and 0.5 ml of stannous chloride were added and the content was diluted to 50 ml using distilled water. After 5 minutes the developed color intensity was measured at 690 nm using spectrophotometer. Finally the concentration of unknown sample with different concentrations (ppm) were calculated from the standard graph.
The Nitrate chemical species is usually monitored for environmental protection purposes in agricultural, food controls, the control of multiple uses of water and checks its portability. Due to the formation of carcinogenic N-nitrosamines and also essential to indicate the organic pollution in water. The determination of the exact concentration of nitrate is required. In recent periods a growing interest in the determination of nitrate levels in food products, it has been observed fundamentally owing to the potential reduction of nitrate to nitrite and have adverse effects on various living organisms including humans and animals. The analysis was performed using nitrate solution, about 0.25 ml sample solution is pipette out into a 50 ml beaker and to that add 0.8 ml of 5% (w/v) salicylic acid in conc. H2SO4. Later 20 minutes, add 19 ml NaOH and this is to raise the pH above 12. Then the samples with different concentrations (ppm) were allowed to cool and measured the absorbance in terms of optical density (OD) at 410 nm using a UV-Visible spectrophotometer. The initial and residual concentration of nitrate in terms of optical density (OD) value was measured using UV-Visible spectrophotometer.
The sulfates chemical species are discharged into water from the sources of mines and smelters, kraft pulp and paper mills, textile mills and tanneries. The elements include sodium, potassium and magnesium sulfates are all highly soluble in water. While calcium, barium sulfates and many heavy metal sulfates are less soluble. The atmospheric sulfur dioxide formed by the combustion of fossil fuels and from metallurgical roasting processes may also contribute to the sulfate content of surface waters. The sulfur trioxide was produced by the photolytic or catalytic oxidation of sulfur dioxide combines with water vapor to form dilute sulfuric acid (which cascades as “acid rain”). The sulphate ion is precipitated in hydrochloric acid medium with barium chloride (BaCl2) so as to form barium sulphate (BaSO4) crystals of uniform size. Light absorbance by BaSO4 suspension is measured by a spectrophotometer. To the series of standards, 5.0 ml of conditioning reagent was added and then mixed thoroughly using stirring apparatus. While stirring and a spoon full of BaCl2 crystals was added and stirred for one minute at constant speed. Immediately after stirring period has ended, the absorbance of the solution was measured spectrophotometrically at 420 nm. The sulphate concentration in the unknown sample is estimated by running the samples (50 ml) through the entire procedure. The different concentrations (ppm) of SO4 in the sample is obtained from the absorbance of samples using the calibration curve.
The water samples from different concentrations (ppm) were filtered using filter paper. Based on Shubham Jain (2017) approach, 5 ml of sample water was taken and add 5 ml of S reagent was added to each concentration of water sample and make up to 30 ml using distilled water. The water sample was measured using UV-Vis spectrometer at 544 nm. The Standard concentration of fluoride was also taken before the treatment of plant. The spectrophotometric approaches are extensively used in the determination of fluoride because of advantages includes simplicity, convenience, accuracy and reproducibility (Zolgharnein, et al., 2009). They are based on the reaction of fluoride with colored metal chelate complexes, producing either a mixed ligand ternary complex or replacement of the ligand by fluoride to give a colorless metal-fluoride complex and the free ligand with a color different to the metal-ligand complex (Einaga and Iwasaki, 1981).
Estimation of chlorophyll content of duckweed plants
The chlorophyll concentration of leaf is a significant parameter that is commonly measured as an indicator of chloroplast content of the plant, its photosynthetic mechanism and the plant metabolism. The chlorophyll is an antioxidant compounds which are present and stored in the chloroplast of green leaf plants and principally present in the green area of leaves, stems, flowers and their roots (Srichaikul, et al., 2011).
Take one gram of leaf sample, then it was finely cut into pieces which then gently grind with a clean pestle and mortar. To this homogenized leaf material was added with 20 ml of 80% acetone and 0.5 gm MgCO3 powder. Then the materials were further grind gently in to fine samples. These sample was then put into a refrigerator at 40°C for 4 hours and subsequently the sample was centrifuged at 500 rpm for 5 minutes. The supernatant was then transferred to 100 ml volumetric flak and the final volume was made up to 100 ml with addition of 80% acetone. The color absorbance of the solution was estimated by a spectrophotometer using at 645 nm and 663 nm wavelength against the solvent. The acetone (80%) was used as a blank (APHA, 1989). The leaf material are crushed using mortar and pest leaded acetone and MgCO3, kept for 4 hours in freeze at 4°C and centrifuge at 500 rpm for 5 minute then measured absorbance on spectrophotometer.
Estimation of biomass of duckweed Plant
The measurement of fresh and direct biomass after 15th day of the phytotreatment experiments, a L. minor (duck weed) plants in each individual replicate were harvested and rinsed with distilled water to remove any ions adhering to plants surface and dried out by blotting on filter papers separately, then the both the fresh and dry biomass was weighed and they are measured subsequently.
Results and Discussion
Eutrophic wastewater characterization
From Fig. 3, represented the characteristics of synthetic wastewater used in the study, it was observed that the following parameters.
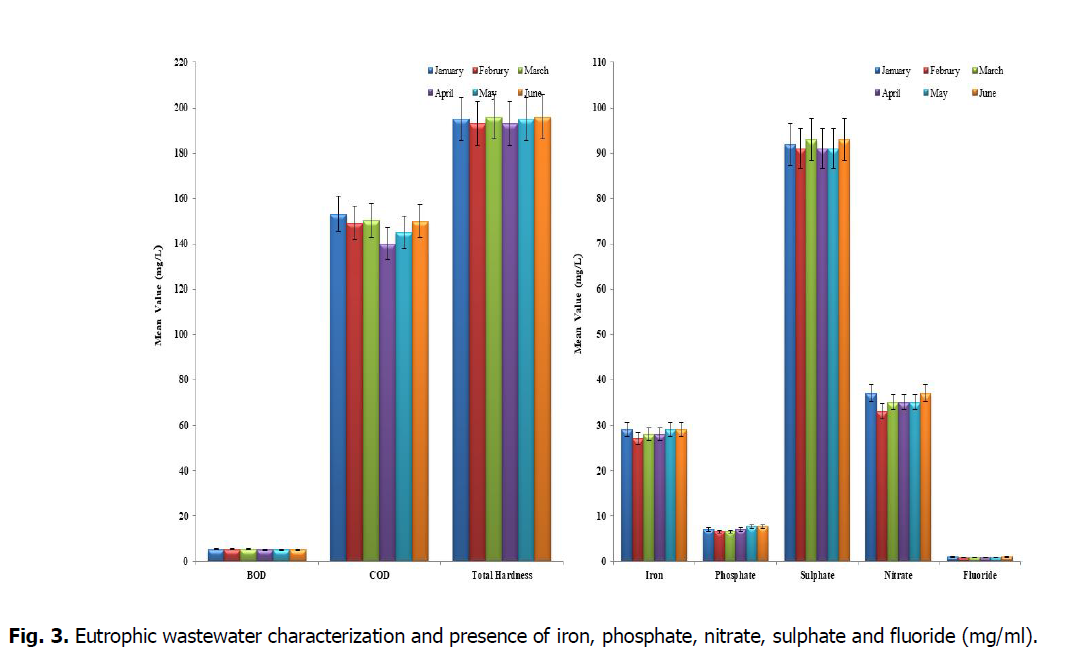
Fig. 3. Eutrophic wastewater characterization and presence of iron, phosphate, nitrate, sulphate and fluoride (mg/ml).
The biological oxygen demand (BOD) and chemical oxygen demand (COD) are measured of the organic matter present in water, the values of BOD and COD in this study were found to be within the permissible limits (value) in all the samples. And these values were higher in seasonal variation. So the demand for oxygen in all the lakes is more. This results from the addition of sewage in to the lake waters at all the times.
The hardness formed by multivalent metallic cations that are capable of reacting with soap to form precipitates and along with certain anions present in the water leads to scale form. The hardness causing cations includes the divalent calcium, magnesium, strontium, ferrous and manganous ions. The hardness of water reflects the nature of the geological formation when it is in contact. The hardness level should be within 200 mg/L for drinking purpose. The lake showed the higher total hardness than the other water bodies. The total hardness exceeding 300 mg/L is generally not recommended for drinking as reported by Srinivasa Rao and Nageswara Rao (2010). Due to more evaporation and low inflow of water in summer season, the total hardness was found to be more in summer than winter. The pH determines whether the water is acidic or alkaline or neutral and its permissible limit for freshwater is 6.5-9.2 (Hazir and Çadraku, 2021). All the samples were found to have pH values within the permissible limit.
Bioremoval of iron, phosphate, nitrate, sulphate and fluoride by Phytotreatment wastewater from Eutrophic Lake using L. minor
Iron is a micronutrient present in water bodies, the presence of iron in water can be attributed to the suspension of the rocks and minerals, landfill leachates, sewage and industrial effluents. According to the study by Ayers and Westcot (1976), the limit of iron should be within 5.0 mg/L. In both the seasons, the amount of iron present in the lake samples was 28 mg/L. So it will disturb the property of water and also effects the aquatic and nearby environment. Phosphate may be due to the high rate of decomposition of waste materials or due to surface run-off from the surrounding crop fields, evaporation of water and low water level during summer. The phosphate content during winter was higher and lower in summer. The sulphate may be present naturally in water bodies and due to bathing and washing of clothes as revealed by Jain, et al., (1996). The limit for sulphate content is 200-400 mg/L. All the water samples were found to contain sulphate levels below the lower limit during winter and summer seasons. The nitrates and nitrites are the most abundant forms of dissolved nitrogen in groundwater and surface water due to agricultural and domestic activities. The high concentration of nitrates can stimulate the growth of aquatic plants and may be a health hazard to juvenile mammals. In present study, the nitrite content was within the permissible limit for all the four samples in winter and slightly increased in the summer. But the values were below the maximum acceptance level. The low nitrate concentration may be due to biological destruction of self-purification properties of water bodies. The fluoride ion is one of the inorganic anions in water which leads to teeth problems when present above the acceptable limit. The present study has established that all the four water samples have their fluoride content below acceptable limit in both winter and summer seasons.
The Fig. 4, showed that the bioremoval of iron in the concentrations of 10, 20 and 40 ppm shows high efficiency of percentage removal as 98%, 95.8% and 93.7% at 510 nm when compared to the concentrations of 60, 80 and 100 ppm. So, the concentration from 10 ppm to 50 ppm is advisable concentration in the Kurichi lake water whereas above 60 ppm plants i.e., L. minor cannot with stand in these concentrations so the concentration above 60 ppm in the Kurichi Lake water is not advisable. From the figure, it was observed that the L. minor at concentrations 10, 20 and 40 ppm are grown well, having good growth whereas the plants in the concentrations of 60, 80 and 100 ppm are slowly showed their growth inhibition and started to decay respectively.
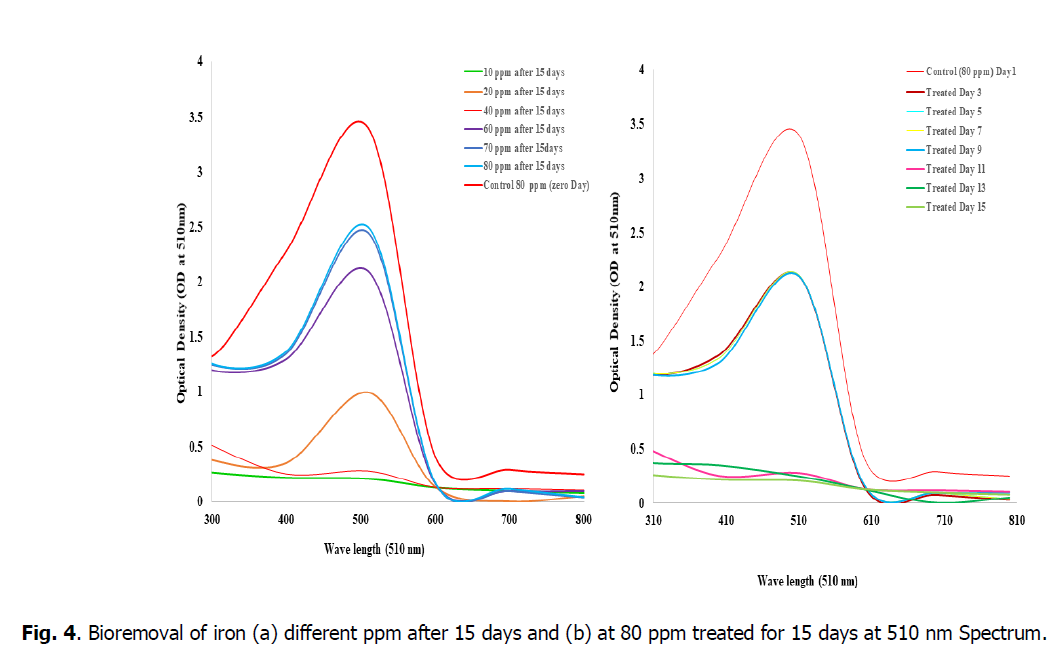
Fig. 4. Bioremoval of iron (a) different ppm after 15 days and (b) at 80 ppm treated for 15 days at 510 nm Spectrum.
The microbial-mediated Fe3+ reduction was the key for the initiation of the redox cycle. The removal mechanism of nitrate was the similar as that of nitrite, i.e., abiotic oxidization of biogenic Fe2+ coupled with the reduction in NO3/NO2 was the sole process responsible for the removal of total nitrogen (TN). The difference was that in the nitrate system particularly after the third addition of nitrate, reduction of iron minerals can no longer be observed due to the accumulation of the intermediate product nitrite from nitrate reduction. The biogenic Fe2+ were formed by the microbial-mediated Fe3+ reduction reaction and it reacted with nitrite to remove from the system. The time for this reaction may be intensified to complete the removal of nitrite before the adding of further nitrate. The overall is the excellent of Fe3+/Fe2+ cycling of the two iron oxides tested are potential application for the continuous nitrate bioremoval in the aquatic environment.
In the earlier reports, the change of ferrihydrite and magnetite throughout redox cycling of nitrate was measured by X-Ray Diffraction study (XRD). The XRD pattern after 170 h reaction of ferrihydrite showed the characteristic peak of magnetite. The results demonstrated that ferrihydrite was slowly converted into magnetite during the process of microbial-mediated Fe3+ reduction. The magnetite remained structurally consistent throughout and Fe2+ obtained by the reduction of structural state Fe3+ by iron-reducing bacteria which would undergo a series of complex reactions with iron (hydrogen) oxides including the process of adsorption, electron transfer, reduction dissolution, atomic exchange and crystal phase recombination and thus transformed to secondary minerals (Zachara, et al., 2002). The biogenic Fe2+ was oxidized and reacted with the added NO3-N that results in completing one iron reduction-oxidation (redox) cycle. Hence it was the biotic and biogenic reduction of Fe3+ that started the iron redox cycle and the reaction equations of these redox cycles were reliable with the previous available research work (Lu, et al., 2017). Among these iron-reducing bacteria are the most widely used was the Geobacter sp and Shewanella sp (Lovley, et al., 2004). The iron biological reduction (bio-reduction) process is reasonably slow and it is important to add electron transports to accelerate the existence of the iron bio-reduction process (Zhou, et al., 2016; Yang, et al.,2019). It was also reported that S. oneidensis MR-1 bacteria secrete electron transports (Peretyazhko, et al., 2010). The authors, Li and his colleagues were also observed that high concentrations of nitrate as electron acceptors can inhibit the bio-reduction of iron minerals (Li, et al., 2015). The result indicated that the biotic process attained multiple removal cycles over an extended period of time.
Bioremoval of Fe using Phytotreatment for 15 days
The Fig. 5a, showed that the removal percentage of iron (after 14 days) in the concentrations of 10 and 20 ppm showed high efficiency of percentage removal as 98.7%, and 95.8% respectively at 510 nm when compared to the concentrations of 60, 80 and 100 ppm. So the concentration from 10 ppm to 30 ppm (after 15days) was advisable concentration in the Kurichi Lake water whereas the above 60 ppm plants i.e., L. minor cannot withstand in these concentrations. So the concentration above 60 ppm in the Kurichi Lake water is not advisable. From the Fig. 8, it was observed that the L. minor in the concentrations 10, 20 and 40 ppm are health concerns whereas the plants in the concentrations of 60, 80 and 100 ppm were decayed respectively. From the Fig. 5b, it was observed that at initial period the pH was found to be 7 for the standards of different concentrations 10, 20, 40, 60, 80 and 100 ppm, after the removal of iron from the Lemnna minor and the final pH was found to be reduced (in the range of 8-5.9) for different concentrations (8, 7.7, 7.5, 6.9, 6.2 and 5.9) respectively.
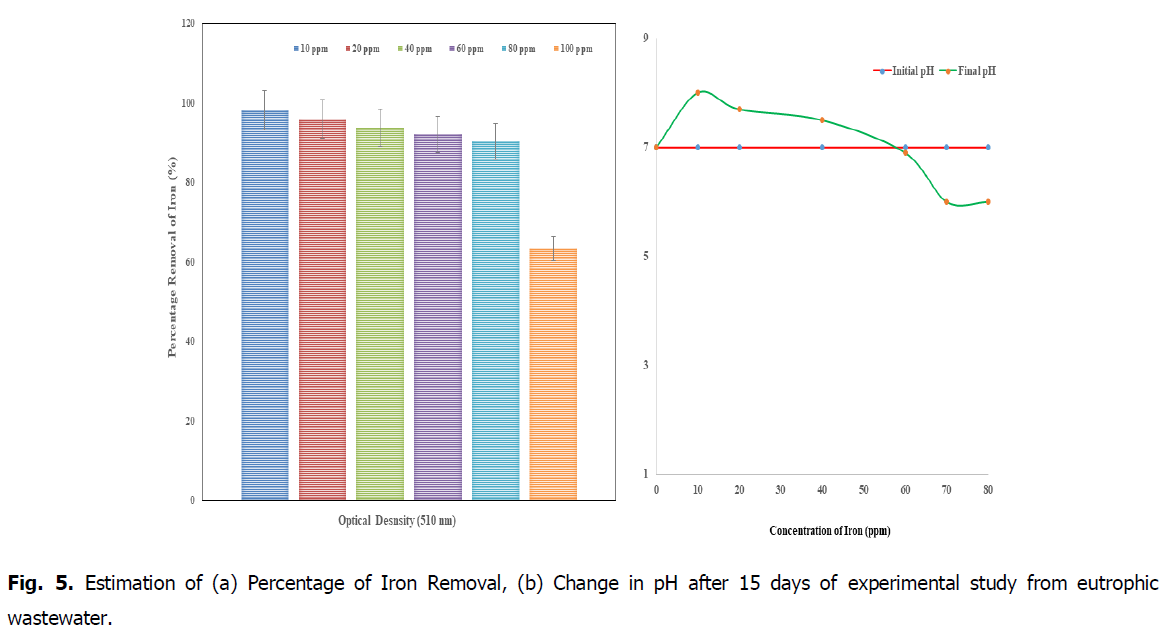
Fig. 5. Estimation of (a) Percentage of Iron Removal, (b) Change in pH after 15 days of experimental study from eutrophic wastewater.
The complete mechanism behind acid mine drainage can be explained by a series of chemical reactions. In the first step ferrous sulphide (pyrite) reacts with water and oxygen from the environment to form ferrous sulphate and sulfuric acid (Egon, et al., 2016). Ferrous sulphate is formed as a result of oxidation of pyrite and may be oxidized even further in an accelerated manner to ferric sulphate in the presence of bacteria like Thiobacillus (Gerardo, et al., 2019). Further the ferric sulphate gets dissolved in the acidic water. Upon contact with water can undergo hydrolysis reaction to form ferric hydroxide with release of hydrogen ions and the hydrogen ions which lead to increase in the acidity of water. The ferric hydroxide formed during this reaction further reacts with pyrite to produce more amount of acid along with ferrous and sulphate ions. The increase in acidity is directly proportional to the oxidation of iron. The following reactions were take place.
FeS2+H2O+3½ O2 → FeSO4+H2SO4
FeSO4 → Fe2(SO4)3 → 2Fe3++ 3SO4-2 Fe3+
Fe3++3H2O → Fe(OH)3+3H+
In wastewater treatment, at high pH, the oxidized iron due to less solubility gets precipitated at the base of the drainage, leaving behind the sulphate content constant. This sulphate content can be used to estimate the acidity of the drainage water. But, with the accumulation of acid, the solubility of iron increases as the pH falls below 3.
The Fig. 6 and Fig. 7, showed that the removal percentage of Phosphate, Nitrate, Sulphate and Fluoride, afte 15 days of phytotreatment in the concentrations of up to 80 ppm showed high efficiency percentage of removal respective elements. The phosphate removal (at 690 nm), was observed as maximum 99% at 10 ppm and minimum 93% at 100 ppm, the nitrate removal (at 410 nm) was observed as maximum 98.7% at 10 ppm and minimum 93% at 100 ppm, the Sulphate removal (at 420 nm) was observed as maximum 98% at 10 ppm and minimum 93% at 100 ppm and the fluoride removal (at 544 nm) was observed as maximum 88% at 10 ppm and minimum 70% at 100 ppm. So the concentration of minimum 10 ppm (after 15 days) was advisable concentration in the Kurichi Lake water whereas the above 60 ppm concentrations, the plants i.e., L. minor cannot withstand. So the concentration above 60 ppm in the Kurichi Lake water is not advisable. From the Fig. 8, it was observed that the L. minor in the concentrations upto 60 ppm are showed good growth after phytotreatment for 15 days’ time period. Whereas the plants in the concentrations of above 60 ppm were slowly started decay in their growth and above 100 ppm they are in death phase of growth occurred.
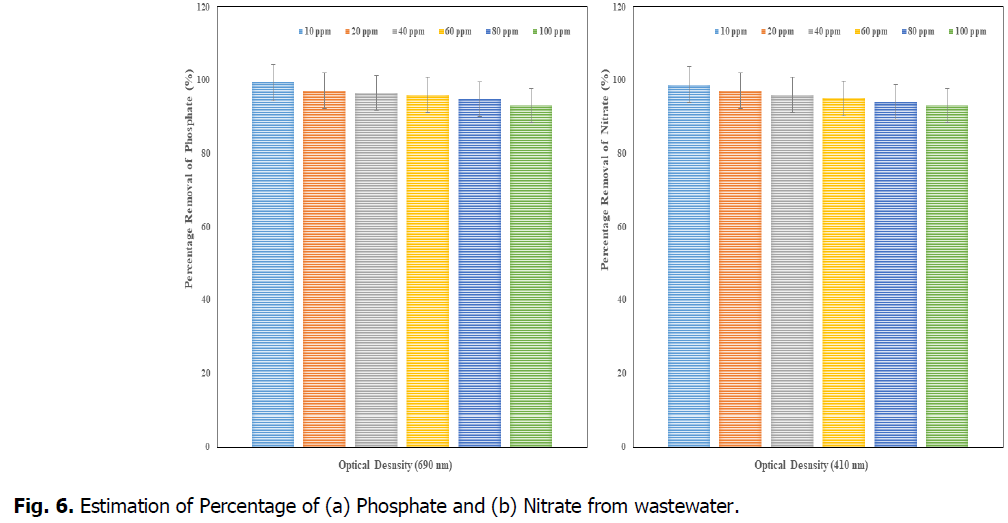
Fig. 6. Estimation of Percentage of (a) Phosphate and (b) Nitrate from wastewater.
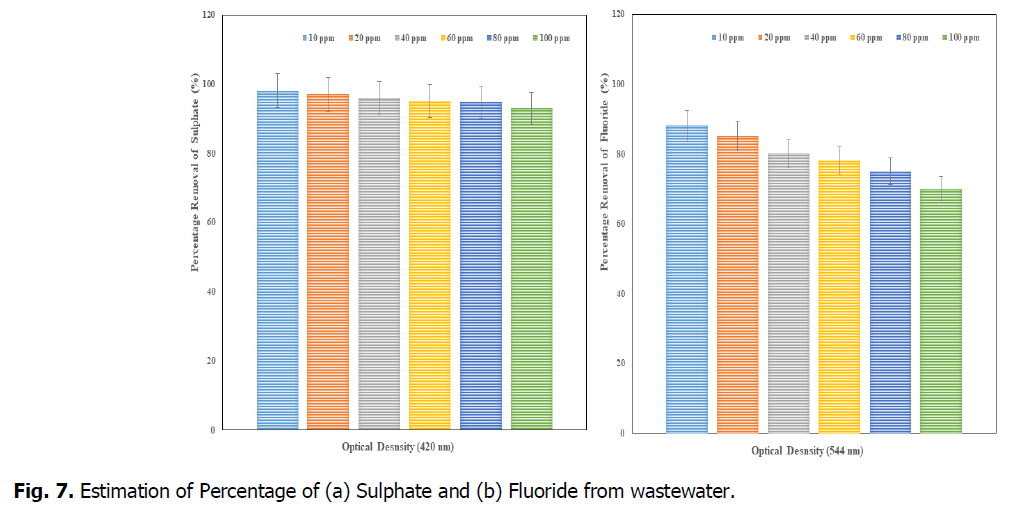
Fig. 7. Estimation of Percentage of (a) Sulphate and (b) Fluoride from wastewater.
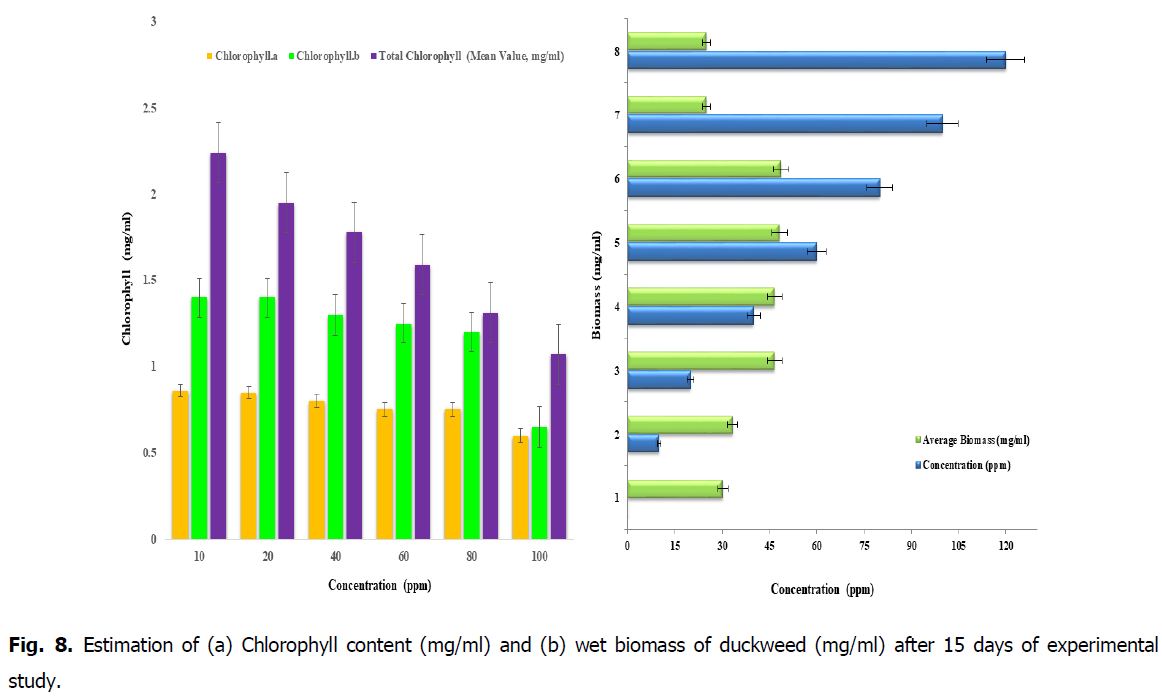
Fig. 8. Estimation of (a) Chlorophyll content (mg/ml) and (b) wet biomass of duckweed (mg/ml) after 15 days of experimental study.
Estimation of biomass on duckweed plants and chlorophyll content
Initially at the start of the phytoremediation process, it was noted that 15 grams of duckweed has been weighed and has been transferred to the each concentrations of 10, 20, 40, 60, 80, 100 and 120 ppm. From the Fig. 8, it was found that after the treatment period of 15 days of removal of iron from the duckweed L. minor the weight of the biomass of the duckweed has been decreased with respect to the concentration of Iron and its retention time. In conclusion, due to the intake of toxicity of iron in the plants respective dosage. From this Fig. 8, it’s was observed that the effect of iron on chlorophyll content after the period of 15 days of time, the chlorophyll content in the concentration 10, 20, 40 and 60 ppm is high when compared to the concentrations of 80 and 100 ppm. It is due to the high biomass content in the concentrations below 60 ppm, therefore this will be the tolerant level of known weight of biomass (Fig. 8).
From the Fig. 8, the experimental study after 15 days showed that gradual reduction of chlorophyll and cell death occurs due accumulation of nutrients, the biosorption and loading of nutrients in batch scale study. The variance between the chlorophyll content in the young and adult leaves of same plant species were observed. It is important to do this kind of study to know the photosynthetic activity of physiological changes of young and adult leaves of the same plants.
The study was also to analyze chlorophyll a and b contents in young and adult leaf of selected plants. Though, the chlorophyll is very significant macromolecule that indicates the photosynthesis activity and energy utilization rate. Moreover it gives us energy in the form of food or plant material. The chlorophyll endures antioxidant properties that can be wide application for discovery of medicinal drugs. It was observed that the chlorophyll content in young and adult leaves and its interface with other macromolecules.
However the chlorophyll production is primarily hinge on permeation of sun light as a chief source of energy for plant (Srichaikul, et al., 2011). In the lab, it was usually estimated by Total Chlorophyll content, from the plant leaves, using pestle and mortar to extract the chlorophyll pigments using acetone, an organic solvent or dimethyl formamide (Arnon, 1949; Porra, et al., 1989; Ling, et al., 2011). The total chlorophyll consists of chlorophyll-a and Chlorophyll-b, these are the essential pigments of the plant photosystems (Richardson, et al., 2002). The primary photosynthetic pigment in plants is chlorophyll a helps to produce energy in plant (Srichaikul, et al., 2011).
Though the chlorophyll “a” content was twice or thrice times higher than that of secondary chlorophyll “b” in plants as reported by Srichaikul, et al., 2011. To obtain the ratio of chlorophyll a and b, the interpretations must be observed and measured the absorption taken at 650 nm wavelength, which was in between the absorption maxima of both (Arnon, 1949, Porra, et al., 1989 and Devmalkar, et al., 2014). The green plants have diverse characters since of the presence of several pigments like chlorophyll, carotenoid, other pigments along with water content together constitute the spectral characteristics of a plant body (Jan-Chang Chen and Chaur-Tzuhn Chen, 2006). The difference in leaf chlorophyll content can delivered information about the physiological condition of a leaf or plant. The critical approaches of leaf chlorophyll content quantification contain traditional technique by means of extraction and spectrophotometric or HPLC analytical instrumentation techniques.
Conclusion
The L. minor were considered to remove iron from wastewater using the bioprocess of phytoremediation technology, so the L. minor (duckweed) used in this study was collected from the eutrophic lake water (Kurichi Lake) and subjected to different concentrations of iron solution (10, 20, 40, 60, 80 and 100 ppm) to determine the efficiency on the removal of iron from waste water. The physio-chemical parameters of Kurichi Lake water such as pH, BOD, COD, iron, sulphate, phosphate, nitrate and fluoride were analyzed and it was observed that the significant physiochemical parameters were founded to be high concentration and the level of iron was considered as concern. Hence, the green sustainable phytotreatment or remediation study was carried out. The L. minor were considered as potential aquatic free floating weed to remove iron from wastewater using the bioprocess of phytoremediation technology and subjected to different concentrations (up to 100 ppm) of iron solution to determine the efficiency on the removal of iron from waste water. At the concentration of 10, 20, 40 and 60 ppm, the removal percentage of iron by L. minor has been efficient when compared to higher concentration of above 60, 80 and 100 ppm. The maximum tolerant level was observed to be maximum at 40-50 ppm, beyond that, the biomass was less and removal efficiency was low. Further the pH, biomass and total chlorophyll content has been observed after 15 days of time period. Further from these observations, indicated that the iron was recorded efficiently removed by L. minor in the lower concentrations (up to 50 ppm) when compared to the higher concentration. So in the Kurichi Lake water, the duckweed can with stand and removed the iron concentrations up to 50 ppm, which effectively remediate the iron pollutant. Hence phytotreatment using L. minor (duckweed) helps and benefits in the remediation of iron from wastewater. This technology affords a simple, cost-effective solution and sustainable ecotechnology which stabilizes the environment with an iron metal pollutant problem bottleneck in public water resources and natural waterways.
References
Ali, A.A., Moustafa, Y.T., Ahmed, S.M., Gomha, S.A. (2020). Duckweed as a biological filter in tilapia fish hatcheries and its impact on tilapia reproduction. Aqua, 13:117-134.
Ajiboye, T.O., Oyewo, O.A., Onwudiwe, D.C. (2021). Simultaneous removal of organics and heavy metals from industrial wastewater: A review. Chemosphere, 262:128379.
Google Scholar, Crossref, Indexed at
APHA. (1998). Standard methods for the examination of water and wastewater. American Public Health Association, Washington, DC.
Apha, A. (2007). WEF (2005) Standard methods for the examination of water and wastewater. American Public Health Association, American Water Works Association, and Water Environment Federation.
Appelo, C.A.J., Van der Weiden, M.J.J., Tournassat, C., Charlet, L. (2002). Surface complexation of ferrous iron and carbonate on ferrihydrite and the mobilization of arsenic. Environmental Science and Technology, 36:3096-3103.
Appenroth, K.J., Krech, K., Keresztes, A., Fischer, W., Koloczek, H. (2010). Effects of nickel on the chloroplasts of the duckweeds Spirodela polyrhiza and Lemna minor and their possible use in biomonitoring and phytoremediation. Chemosphere, 78:216-223.
Google Scholar, Crossref, Indexed at
Arnon, D.I. (1949). Copper enzymes in isolated chloroplasts. Polyphenoloxidase in Beta vulgaris. Plant Physiology, 24:1.
Google Scholar, Crossref, Indexed at
Diro, A., Asere, T.G., Jado, D., Melak, F. (2021). Spectroscopic determination of fluoride using Eriochrome Black T (EBT) as a spectrophotometric reagent from groundwater. International Journal of Analytical Chemistry.
Ayers, R.S., Westcot, D.W. (1985). Water quality for agriculture. Rome: Food and Agriculture Organization of the United Nations, 29:174.
Azimi, A., Azari, A., Rezakazemi, M., Ansarpour, M. (2017). Removal of heavy metals from industrial wastewaters: a review. ChemBioEng Reviews, 4:37-59.
Bolisetty, S., Peydayesh, M., Mezzenga, R. (2019). Sustainable technologies for water purification from heavy metals: Review and analysis. Chemical Society Reviews, 48:463-487.
Bourzama, G., Ouled-Haddar, H., Marrouche, M., Aliouat, A. (2021). Iron uptake by fungi isolated from arcelor mittal-annaba-in the northeast of Algeria. Brazilian Journal of Poultry Science.
Gerba, C.P., Pepper, I.L. (2019). Municipal wastewater treatment. In Environmental and Pollution Science, pp:393-418.
Google Scholar, Crossref, Indexed at
Cao, W., Wang, Z., Ao, H., Yuan, B. (2018). Removal of Cr (VI) by corn stalk based anion exchanger: The extent and rate of Cr (VI) reduction as side reaction. Colloids and Surfaces A: Physicochemical and Engineering Aspects, 539:424-432.
Google Scholar, Crossref, Indexed at
Devmalkar, V.S., Murumkar, C.V., Salunkhe, S.M., Chavan, S.J. (2014). Studies on pigment chlorophyll isolation and estimation of different bryophytes for their biochemical properties. Journal of Natural Product and Plant Resources, 4:56-61.
Dueik, V., Chen, B.K., Diosady, L.L. (2017). Iron-polyphenol interaction reduces iron bioavailability in g complexation to ensure iron bioavailability. Journal of Food Quality.
Dos Santos, E.C., de Mendonça Silva, J.C., Duarte, H.A. (2016). Pyrite oxidation mechanism by oxygen in aqueous medium. The Journal of Physical Chemistry, 120:2760-2768.
Einaga, H., Iwasaki, I. (1981). Spectrophotometric study of the reaction of zirconium with chrome azurol S and fluoride. Talanta, 28:889-900.
Google Scholar, Crossref, Indexed at
El-Beltagi, H.S., Sofy, M.R., Aldaej, M.I., Mohamed, H.I. (2020). Silicon alleviates copper toxicity in flax plants by up-regulating antioxidant defense and secondary metabolites and decreasing oxidative damage. Sustainability, 12:4732.
Google Scholar, Crossref, Indexed at
EPA. (1996). Aquatic plant toxicity test using Lemna spp., tiers I and II. Ecological effects test guidelines. OPPTS 850.4400.
https://cpcb.nic.in/industrial_pollution/New_Action_Plans/CEPI_Action%20Plan_Coimbatore%20.pdf
Fierro, P., Tapia, J., Bertrán, C., Acuña, C., Vargas-Chacoff, L. (2021). Assessment of heavy metal contamination in two edible fish species and water from North Patagonia Estuary. Applied Sciences, 11:2492.
Google Scholar, Crossref, Indexed at
Fritioff, Å., Greger, M. (2003). Aquatic and terrestrial plant species with potential to remove heavy metals from stormwater. International Journal of Phytoremediation, 5:211-224.
Galal, T.M., Gharib, F.A., Ghazi, S.M., Mansour, K.H. (2017). Phytostabilization of heavy metals by the emergent macrophyte Vossia cuspidata (Roxb.) Griff.: A phytoremediation approach. International Journal of Phytoremediation, 19:992-999.
Pineda, G.A.C., Godoy, M.A.M. (2019). Effect of Acidithiobacillus thiooxidans-cysteine interactions on pyrite biooxidation by Acidithiobacillus ferrooxidans in the presence of coal compounds. Brazilian Journal of Chemical Engineering, 36:681-692.
Gorito, A.M., Ribeiro, A.R., Almeida, C.M.R., Silva, A.M. (2017). A review on the application of constructed wetlands for the removal of priority substances and contaminants of emerging concern listed in recently launched EU legislation. Environmental Pollution, 227:428-443.
Google Scholar, Crossref, Indexed at
Miranda, G., Quiroz, A., Salazar, M. (2000). Cadmium and lead removal from water by the duckweed: Lemna gibba L.(Lemnaceae). Hidrobiológica, 10:7-12.
Guittonny-Philippe, A., Petit, M.E., Masotti, V., Monnier, Y., Malleret, L., Coulomb, B., Laffont-Schwob, I. (2015). Selection of wild macrophytes for use in constructed wetlands for phytoremediation of contaminant mixtures. Journal of Environmental Management, 147:108-123.
Google Scholar, Crossref, Indexed at
Çadraku, H.S. (2021). Groundwater quality assessment for irrigation: Case study in the Blinaja river basin, Kosovo. Civil Engineering Journal, 7:1515-1528.
Google Scholar, Crossref, Indexed at
Huang, Y., Wang, L., Wang, W., Li, T., He, Z., Yang, X. (2019). Current status of agricultural soil pollution by heavy metals in China: A meta-analysis. Science of the Total Environment, 651:3034-3042.
Google Scholar, Crossref, Indexed at
Chen, J.C., Yang, C.M., Wu, S.T., Chung, Y.L., Charles, A.L., Chen, C.T. (2007). Leaf chlorophyll content and surface spectral reflectance of tree species along a terrain gradient in Taiwan’s Kenting National Park. Botanical Studies, 48:71-77.
Jia, Z., Li, S., Wang, L. (2018). Assessment of soil heavy metals for eco-environment and human health in a rapidly urbanization area of the upper Yangtze Basin. Scientific Reports, 8:3256.
Joe-Wong, C., Brown Jr, G.E., Maher, K. (2017). Kinetics and products of chromium (VI) reduction by iron (II/III)-bearing clay minerals. Environmental Science and Technology, 51:9817-9825.
Koźmińska, A., Wiszniewska, A., Hanus-Fajerska, E., Muszyńska, E. (2018). Recent strategies of increasing metal tolerance and phytoremediation potential using genetic transformation of plants. Plant Biotechnology Reports, 12:1-14.
Ling, Z., Shi,Z, Xu, S., Dong, M. (2019). Temporal and spatial distributions of nutrients and pollution risk assessment of Taxodium 'zhongshanshan' wetland of Dianchi Lakeside, Southwest of China. Applied Ecology and Environmental Research. 18:1161-1174.
Liu, H., Chen, Z., Guan, Y., Xu, S. (2018). Role and application of iron in water treatment for nitrogen removal: A review. Chemosphere, 204:51-62.
Google Scholar, Crossref, Indexed at
Lovley, D.R., Holmes, D.E., Nevin, K.P. (2004). Dissimilatory fe (iii) and mn (iv) reduction. Advances in Microbial Physiology, 49:219-286.
Marsidi, N., Hasan, H.A., Abdullah, S.R.S. (2018). A review of biological aerated filters for iron and manganese ions removal in water treatment. Journal of Water Process Engineering, 23:1-12.
Google Scholar, Crossref, Indexed at
Mesa, J., Mateos-Naranjo, E., Caviedes, M.A., Redondo-Gómez, S., Pajuelo, E., Rodríguez-Llorente, I.D. (2015). Endophytic cultivable bacteria of the metal bioaccumulator Spartina maritima improve plant growth but not metal uptake in polluted marshes soils. Frontiers in Microbiology, 6:1450.
Moldovan, A., Hoaghia, M.A., Török, A.I., Roman, M., Mirea, I.C., Barabas, R., Cadar, O. (2021). Spatial variation of water chemistry in aries river catchment, western Romania. Applied Sciences, 11:6592.
Google Scholar, Crossref, Indexed at
Myers, C.R., Nealson, K.H. (1988). Bacterial manganese reduction and growth with manganese oxide as the sole electron acceptor. Science, 240:1319-1321.
Olawale, S.A. (2021). Biosorption of heavy metals from aqueous solutions: An insight and review. Archives of Industrial Engineering Review Article, 3:1-31.
Peretyazhko, T.S., Niles, P.B., Sutter, B., Morris, R.V., Agresti, D.G., Le, L., Ming, D.W. (2018). Smectite formation in the presence of sulfuric acid: Implications for acidic smectite formation on early Mars. Geochimica et Cosmochimica Acta, 220:248-260.
Google Scholar, Crossref, Indexed at
Porra, R.J., Scheer, H. (2019). Towards a more accurate future for chlorophyll a and b determinations: The inaccuracies of Daniel Arnon’s assay. Photosynthesis Research, 140:215-219.
Prashanth, L., Kattapagari, K.K., Chitturi, R.T., Baddam, V.R.R., Prasad, L.K. (2015). A review on role of essential trace elements in health and disease. Journal of Dr. NTR University of Health Sciences, 4:75-85.
Qasem, N.A., Mohammed, R.H., Lawal, D.U. (2021). Removal of heavy metal ions from wastewater: A comprehensive and critical review. Npj Clean Water, 4:36.
Rajeshkumar, S., Liu, Y., Zhang, X., Ravikumar, B., Bai, G., Li, X. (2018). Studies on seasonal pollution of heavy metals in water, sediment, fish and oyster from the Meiliang Bay of Taihu Lake in China. Chemosphere, 191:626-638.
Google Scholar, Crossref, Indexed at
Richardson, A.D., Duigan, S.P., Berlyn, G.P. (2002). An evaluation of noninvasive methods to estimate foliar chlorophyll content. New Phytologist, 153:185-194.
Schneider, L., Mariani, M., Saunders, K.M., Maher, W.A., Harrison, J.J., Fletcher, M.S., Haberle, S.G. (2019). How significant is atmospheric metal contamination from mining activity adjacent to the Tasmanian Wilderness World Heritage Area? A spatial analysis of metal concentrations using air trajectories models. Science of The Total Environment, 656:250-260.
Google Scholar, Crossref, Indexed at
Srichaikul, B., Bunsang, R., Samappito, S., Butkhup, S., Bakker, G. (2011). Comparative study of chlorophyll content in leaves of Thai Morus alba Linn. Species. Plant Science Research, 3:17-20.
Srinivasa, R., Nageswara, R. (2010). Assessment of groundwater quality using water quality index. Archives of Environment and Science, 7:1-5
Su, L., Nan, B., Craig, N.J., Pettigrove, V. (2020). Temporal and spatial variations of microplastics in roadside dust from rural and urban Victoria, Australia: implications for diffuse pollution. Chemosphere, 252:126567.
Google Scholar, Crossref, Indexed at
Su, P., Zhang, J., Tang, J., Zhang, C. (2019). Preparation of nitric acid modified powder activated carbon to remove trace amount of Ni (II) in aqueous solution. Water Science and Technology, 80:86-97.
Syukor, A.A., Sulaiman, S., Siddique, M.N.I., Zularisam, A.W., Said, M.I.M. (2016). Integration of phytogreen for heavy metal removal from wastewater. Journal of Cleaner Production, 112:3124-3131.
Google Scholar, Crossref, Indexed at
The Environmental Protection Agency. 2019. Drinking water regulations and contaminants.
Usharani, K., Keerthi, K.V. (2020). Nitrate bioremoval by phytotechnology using Utricularia aurea collected from eutrophic lake of Theerthamkara, Kerala, India. Pollution, 6:149-157.
Usharani, K., Divya, K., Sruthilaya, K. (2020). Combined effect of nitrate bioremoval by aquatic free floating plant an association with filamentous cyanobacteria.
Usharani, K., Sruthilaya, K., Divya, K. (2017). Determination of nitrate utilization efficiency of selective strain of Bacillus sp. isolated from Eutrophic Lake, Theerthamkara, Kasaragod, Kerala. Pollution, 3:55-67.
Usharani, K., Lakshmanaperumalsamy, P., Muthukumar, M. (2013). Optimization of phosphate removal from synthetic wastewater by bacterial consortium using Box-Behnken design. Environmental Engineering and Management Journal.
Usharani, K., Lakshmanaperumalsamy, P. (2010). Studies on the removal efficiency of phosphate from wastewater using Pseudomonas sp YLW-7 and Enterobacter sp KLW-2. Global Journal of Environmental Research, 4:83-89.
Krishnaswamy, U., Muthuchamy, M., Perumalsamy, L. (2011). Biological removal of phosphate from synthetic wastewater using bacterial consortium.
Krishnaswamy, U., Muthusamy, M., Perumalsamy, L. (2009). Studies on the efficiency of the removal of phosphate using bacterial consortium for the biotreatment of phosphate wastewater. European Journal of Applied Sciences, 1:6-15.
Velichkova, K.N., Sirakov, I.N. (2013). The usage of aquatic floating macrophytes (Lemna and Wolffia) as biofilter in recirculation aquaculture system (RAS). Turkish Journal of Fisheries and Aquatic Sciences.
Wang, J., Xie, Q., Li, A., Liu, X., Yu, F., Ji, J. (2020). Biosorption of hexavalent chromium from aqueous solution by polyethyleneimine-modified ultrasonic-assisted acid hydrochar from Sargassum horneri. Water Science and Technology, 81:1114-1129.
World Health Organization. (2017). Guidelines for drinking-water quality: first addendum to the fourth edition.
Lu, Y., Liu, H., Huang, X., Xu, L., Zhou, J., Qian, G., Chen, X. (2021). Nitrate removal during Fe (III) bio-reduction in microbial-mediated iron redox cycling systems. Water Science and Technology, 84:985-994.
Zachara, J.M., Serne, J., Freshley, M., Mann, F., Anderson, F., Wood, M., Myers, D. (2007). Geochemical Processes Controlling Migration of Tank Wastes in Hanford's Vadose ZoneAll rights reserved. No part of this periodical may be reproduced or transmitted in any form or by any means, electronic or mechanical, including photocopying, recording, or any information storage and retrieval system, without permission in writing from the publisher. Vadose Zone Journal, 6:985-1003.
Zgłobicki, W. (2022). Special issue on heavy metals in the environment-causes and consequences. Applied Sciences, 12:835.
Google Scholar, Crossref, Indexed at
Zhang, X.H., Liu, J., Huang, H.T., Chen, J., Zhu, Y.N., Wang, D.Q. (2007). Chromium accumulation by the hyperaccumulator plant Leersia hexandra Swartz. Chemosphere, 67:1138-1143.
Google Scholar, Crossref, Indexed at
Zhang, Y., Duan, X. (2020). Chemical precipitation of heavy metals from wastewater by using the synthetical magnesium hydroxy carbonate. Water Science and Technology, 81:1130-1136.
Author Info
K. Usharani1,2* and V. Arunkumar12Department of Civil and Environmental Engineering, School of Engineering, Sao Paulo State University, UNESP, Bauru, SP, Brazil
Citation: Usharani, K., Arunkumar, V. (2023). Bioremoval and resource recovery of nutrients by phytoremediation using aquatic free floating plants Lemna minor. Ukrainian Journal of Ecology. 13: 13-27.
Received: 01-May-2023, Manuscript No. UJE-23-105572; , Pre QC No. P-105572; Editor assigned: 03-May-2023, Pre QC No. P-105572; Reviewed: 15-May-2023, QC No. Q-105572; Revised: 22-May-2023, Manuscript No. R-105572; Published: 29-May-2023, DOI: 10.15421/2023_449
Copyright: This is an open access article distributed under the terms of the Creative Commons Attribution License, which permits unrestricted use, distribution, and reproduction in any medium, provided the original work is properly cited.